Our Planet’s Vanishing Legacy
The world is experiencing biodiversity loss at an unprecedented rate. In 2024, scientists estimated that 27K species were disappearing each year, orders of magnitude above the natural background rate of up to 100 species per year. That same year, the World Wildlife Fund (WWF) reported a 73% decline in monitored wildlife populations over the last half century. This builds on projections from 2019 indicating a disappearance of 30-50% of all animal species by 2050, a decline representing not just a loss of unique lifeforms but a collapse of the complex ecological relationships evolved over millions of years.
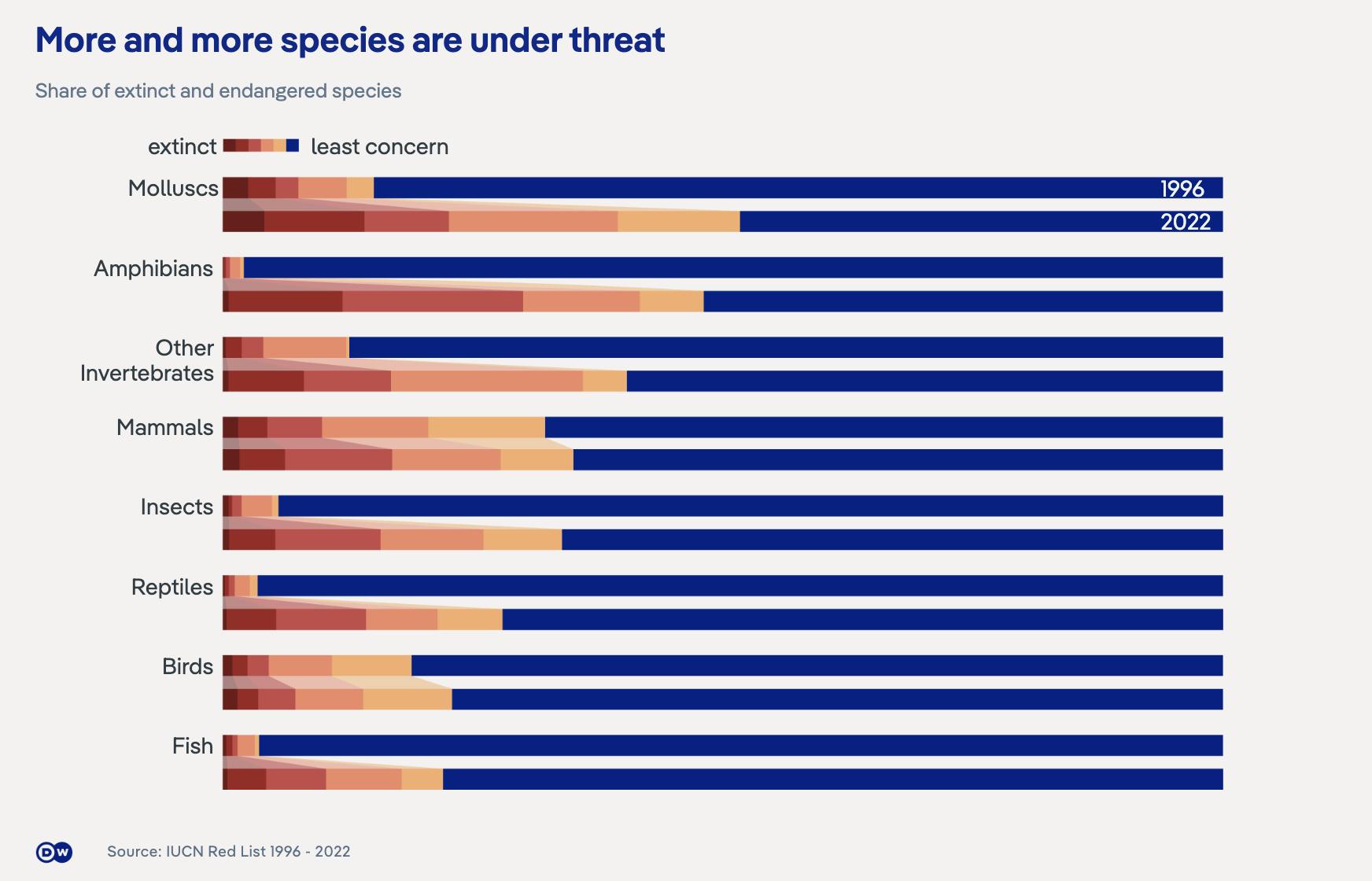
Source: DW
The numbers tell a stark story: we are in the midst of Earth’s sixth mass extinction. But unlike previous catastrophes, there is no asteroid impact, no volcanic eruption, no cataclysm to blame. Instead, we have become the agents of planetary change. In 2023, the Amazon rainforest, home to 10% of known species, had lost nearly 20% of its original territory to cattle ranching, soy production, and illegal logging, pushing it close to a tipping point where it could transform into savanna, releasing billions of tons of stored carbon in the process.
This trend is global. At the beginning of the 21st century, industrial fishing fleets had depleted 90% of large ocean fish populations since 1950, while carbon emissions have increased ocean acidity, dissolving coral reef structures that support 25% of all marine species. As of 2018, scientists project that 90% of the world's coral reefs will be functionally extinct by 2050 without dramatic intervention. Human-driven habitat destruction, climate change, pollution, and overhunting have all contributed to this unfolding crisis, the consequences of which will come to affect us directly.
When habitats degrade, species disappear, ecological networks unravel, and the natural infrastructure underpinning human civilization is put in jeopardy. As of 2019, more than 25% of non-domesticated, livestock-related species and 15% of animal pollinator species face extinction risk, further destabilizing the already threatened state of global food security. By 2020, over 75% of new diseases arose from eroded boundaries between humans and wildlife, with degraded ecosystems forcing closer contact with disease reservoirs. Of all newly approved anti-cancer drugs in the four decades prior to 2019, 25% of them are related to or derived from natural products, a testament to humanity’s continued reliance on evolution’s laboratory. In short, biodiversity loss undermines ecosystem services that humans depend on, from the food supply chain and pollination to medical innovation and disease regulation.
The good news is that loss of biodiversity may not be an irreversible trend, thanks to recent advancements in technology.
From Fiction to Reality: De-Extinction Technology
Traditional conservation approaches, like establishing protected areas, regulating hunting and fishing, and restoring damaged habitats remain vital, but are increasingly insufficient on their own. As climate change forces species to shift their ranges and alters the composition of ecosystems, static protected areas may no longer shelter the species they were designed to protect. Many critically endangered species now have populations too small to maintain genetic diversity, leaving them vulnerable to disease and environmental change even within protected zones.
The collision of crises across extinction, climate change, and ecosystem collapse demands new tools and strategies that can address root causes rather than symptoms. While protected areas and species recovery programs continue to be implemented, they can only prevent further losses, not reclaim what's already gone. This limitation has spurred scientists to ask a once-unthinkable question: What if we could bring extinct species back?
When Michael Crichton published Jurassic Park in 1990, the idea of resurrecting extinct creatures seemed entertaining but scientifically implausible. The novel's premise of extracting dinosaur DNA from amber-preserved insects captured the public imagination but was dismissed by scientists as fantasy. Yet just three decades later, de-extinction has moved from science fiction to scientific possibility, though with methods far different from those imagined by Crichton.
In 2025, headlines about the creation of woolly mice and claims about the rebirth of dire wolves captured public attention. The company behind it, Colossal Biosciences, announced these achievements as part of its de-extinction programs. Founded in 2021 by tech entrepreneur Ben Lamm and geneticist George Church, Colossal Biosciences has raised $448.1 million as of April 2025 to develop technologies for rewilding extinct species. Specifically, the company emphasizes functional de-extinction, or the process of generating organisms that resemble and are genetically similar to extinct species but with enhanced adaptability to today’s environment.
Colossal Biosciences’ business model mirrors NASA’s technology transfer: while the company’s mission remains focused on ecological conservation and restoration, it plans to generate revenue through spinouts of its technology, similar to the inventions developed from space missions (like GPS or memory foam). Unlike Jurassic Park's focus on dinosaurs (which went extinct 65 million years ago), Colossal Biosciences’ projects center around the woolly mammoth, thylacine (Tasmanian tiger), dodo, and dire wolf. It targets these species because they were lost relatively recently, often due to human activity, whose ecological niches remain unfilled. The technological foundations making de-extinction possible rest in three areas of biotechnology that have seen advancements in the past decade.
Next-Generation DNA Sequencing
DNA sequencing is a method for determining the precise order of nucleotides in DNA. The technique has undergone a transformation since the Human Genome Project was completed in 2003, which took 13 years and cost nearly $3 billion to sequence a single human genome. Today, a complete human genome can be sequenced in less than a day for under $1K, in large part thanks to next-generation sequencing (NGS) technology.
However, ancient DNA presents unique challenges beyond those of modern samples. The genetic material in ancient specimens has typically degraded into fragments as short as 40 base pairs, accumulated chemical damage, and become contaminated with environmental and microbial DNA. Recovering viable genetic information from specimens thousands or millions of years old requires specialized techniques.
The core technology behind ancient genome sequencing relies on massive parallelization - examining millions of DNA fragments simultaneously rather than one at a time. Most short-read sequencing platforms use a sequencing-by-synthesis approach, where fluorescently-labeled nucleotides are incorporated into growing DNA strands, imaged, and then cleaved to allow the next base addition. This cycle repeats billions of times, capturing individual sequence "reads" from ancient DNA libraries that act like tiny snapshots of the original genome.
For highly degraded ancient samples, researchers employ specialized library preparation methods, particularly single-stranded library construction techniques. Unlike conventional double-stranded approaches, these methods capture ultrashort DNA fragments that would otherwise be lost an advantage when working with specimens where DNA has broken down over thousands of years. Specialized adapters are attached to both ends of each DNA fragment, allowing amplification of even minute quantities of genetic material.
While short-read sequencing has been the workhorse of paleogenomics, newer long-read sequencing technologies (like PacBio and Oxford Nanopore) are increasingly valuable for ancient DNA work. These platforms generate continuous reads tens of thousands of bases long, helping resolve repetitive regions and structural variants that short reads cannot bridge. However, these methods typically require higher DNA input quantities and better preservation, making them challenging to apply to degraded ancient samples without protocol adaptations.
The final assembly of ancient genomes involves bioinformatic pipelines designed specifically for damaged DNA. Iterative mapping algorithms progressively refine the genome reconstruction, reducing reference bias with each cycle. Post-processing identifies damage patterns typical of ancient DNA (particularly cytosine deamination resulting in C-to-T and G-to-A transitions at fragment ends) and applies appropriate quality filters to ensure reliable results.
For de-extinction projects, the reconstructed paleogenome serves as a blueprint for identifying genetic differences between extinct species and their closest living relatives, potentially enabling ecological resurrection of lost biodiversity through targeted genetic engineering of proxy species. For Colossal Biosciences, this sequencing technology has helped obtain high-quality mammoth, dodo, and thylacine genomes.
Multiplexed CRISPR Gene Editing
Comparing woolly mammoth and Asian elephant genomes reveals millions of genetic differences, including thousands of distinct protein-coding genes. This creates a fundamental challenge that makes traditional genome editing approaches, which modify one gene at a time, practically impossible for de-extinction.
Because of this, Colossal Biosciences relies on multiplex gene editing, technology that can modify multiple genomic sites simultaneously. Rather than recreating a perfect extinct animal or using ancient DNA, the strategy targets key trait-defining genes to modify in modern-day species. For the mammoth project, this means focusing on cold-adaptation traits: smaller ears, dense fur, specialized hemoglobin, and subcutaneous fat deposits.
Scientists estimate that 50+ precise edits will create what they call an "Arctic elephant" (a cold-resistant elephant with core biological traits of the woolly mammoth), while the recently de-extincted dire wolves had 20 edits made to 14 genes in the common gray wolf.
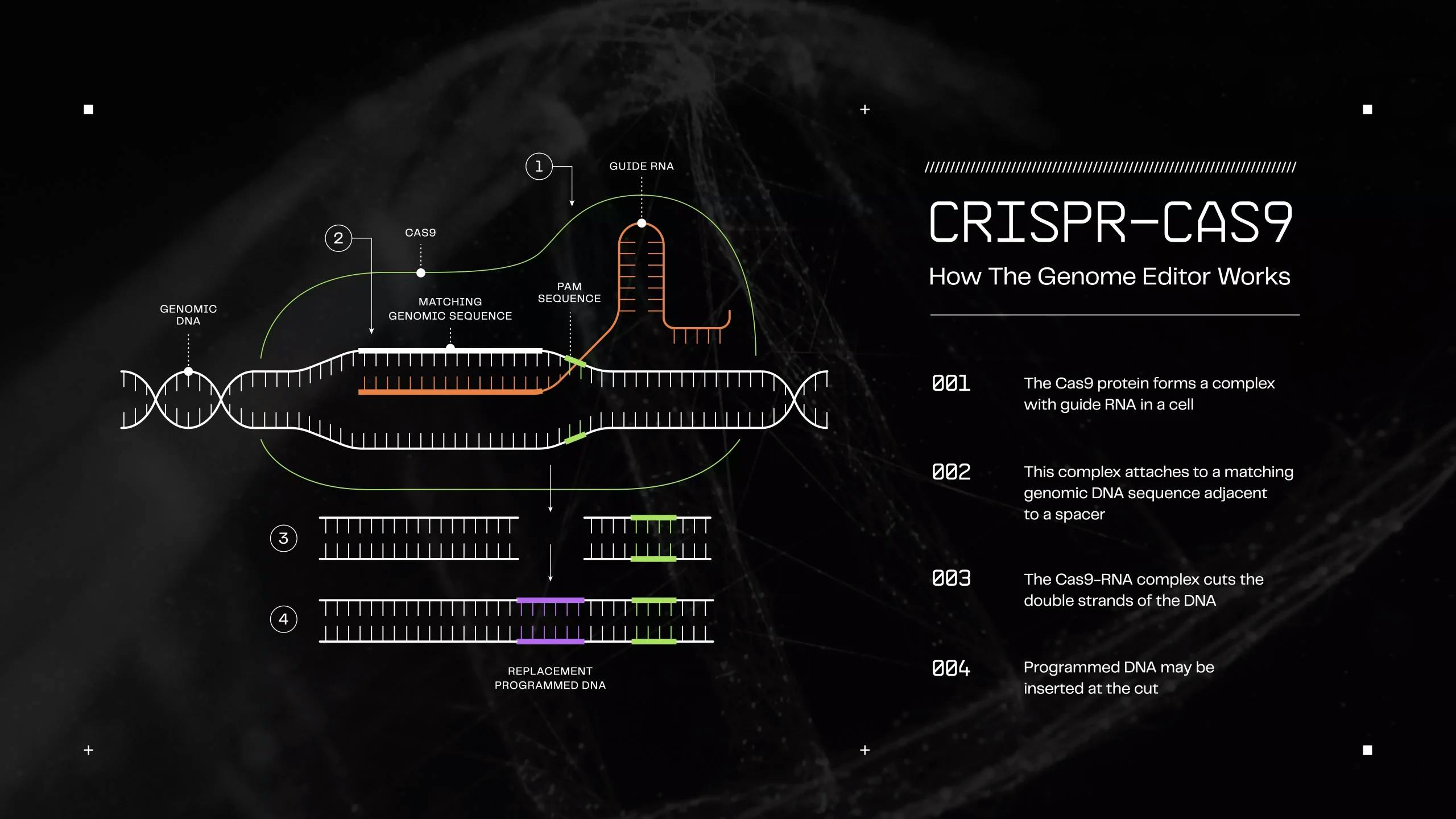
Source: Colossal Biosciences
CRISPR works like molecular scissors guided by a GPS system. The process begins with designing guide RNAs — small RNA molecules that act as the "GPS coordinates" to direct the CRISPR enzyme (like Cas9) to specific locations in the genome. The guide RNA contains a sequence that matches the DNA target site, allowing the Cas9 enzyme to find and bind to exactly where modifications are needed.
Once bound to the target, the enzyme cuts both strands of the DNA at that precise location. This creates a break that the cell's natural repair machinery responds to. Scientists can exploit this repair process in two main ways: by allowing the cell to repair the break imperfectly (which often disrupts gene function) or by providing a template DNA that the cell uses to repair the break, thereby introducing specific changes into the genome.
Multiplex editing works by delivering multiple guide RNAs alongside CRISPR-Cas9 or similar enzymes into cells. Each guide RNA directs the editing machinery to a specific genomic target, enabling concurrent modifications across different chromosomes. CRISPR arrays leverage the natural processing abilities of some Cas enzymes, particularly Cas12a, which can process multiple guides from a single transcript.
However, the efficiency of each edit ranges from 5-80%, and adding more targets reduces overall success rates as cellular repair mechanisms become overwhelmed. Finding the optimal balance between editing ambition and cellular viability represents a technical hurdle. Too few edits per round means the project proceeds at a glacial pace; too many increases the risk of cell death or introduces unintended mutations.
Even with multiplex approaches, the sheer scale of genomic differences means that de-extinction projects can only hope to produce hybrid animals, not true resurrections. As such, Colossal Biosciences has looked to enhance and apply multiplex CRISPR technologies for its approach to functional de-extinction, producing hybrids with selected extinct species traits for future rewilding.
Reproductive Technologies
The final bridge between genetic engineering and living organisms in de-extinction involves reproductive technologies that transform edited cells into viable embryos. For mammoth de-extinction, Colossal Biosciences uses induced pluripotent stem cells (iPSCs) to help bring its hybrid animals to life. iPSCs are created by reprogramming ordinary somatic cells, like skin cells, into a state capable of differentiating into any type of tissue. In March 2024, Colossal Biosciences successfully generated the first-ever elephant iPSCs that carried edited DNA, allowing scientists to study the effects of the extinct species’ traits without requiring living specimens.
After confirming that the genetic edits work properly, Colossal Biosciences uses somatic cell nuclear transfer (SCNT). This involves taking the nucleus from a genetically modified cell and implanting it into an egg cell that has had its nucleus removed. For mammoth de-extinction, Asian elephants serve as the genetic base while African elephants are the potential surrogate mothers. Because of the low success rate of SCNT and the 22-month gestation period for elephants, scientists will likely need to implant dozens of fertile elephants to get one viable birth, which is why endangered Asian elephants cannot serve as surrogates. For the thylacine project, researchers have also achieved progress with marsupial reproductive technologies, including the first artificial techniques that can induce ovulation in fat-tailed dunnarts and grow fertilized single-cell marsupial embryos to over halfway through pregnancy using an artificial uterus.
The dodo project requires an entirely different reproductive approach due to avian biology. Instead of directly manipulating eggs, scientists work with primordial germ cells (PGCs) - the early embryonic cells that eventually become eggs or sperm. PGCs are extracted from the dodo's closest living relative, the Nicobar pigeon, edited to express dodo traits, and injected into developing chicken embryos. These modified chickens would then produce dodo egg and sperm cells. The project also involves creating chimeric chickens to serve as surrogates, since dodo eggs were much larger than Nicobar pigeon eggs, making direct surrogacy impossible.
Beyond De-Extinction: The Biotech Ripple Effect
The technologies powering Colossal Biosciences' de-extinction projects have catalyzed a biotechnological renaissance extending far beyond bringing back mammoths or dodos. Every breakthrough in ancient DNA sequencing, multiplex gene editing, and reproductive technology creates ripple effects across conservation biology, medicine, and agriculture, demonstrating how the pursuit of extinct species will reshape our relationship with the living world.
The mammoth de-extinction project shows how resurrected species could serve as ecological engineers. During the Pleistocene, mammoth herds maintained grasslands that stored more carbon than today's Arctic tundras. By trampling shrubs, compacting snow, and fertilizing soil, mammoths helped maintain permafrost that contains 1.6 billion metric tons of carbon. Colossal Biosciences' de-extincted mammoths could help restore these carbon-trapping ecosystems and slow permafrost melt, introducing an approach to climate change mitigation intertwined with biodiversity restoration through biological solutions.
The same multiplex editing techniques developed for de-extinction are also already being applied to boost genetic diversity in critically endangered populations. As of April 2025, there are fewer than 20 red wolves remaining in North America, all of which are descendants of just 12 founder individuals. To address this dangerous genetic bottleneck, Colossal Biosciences has cloned two litters of red wolves from three distinct cell lines, which would increase the number of founding lineages by 25% if added to the captive breeding population. Similar approaches are being tested for other species close to extinction, including northern white rhinos, with only two females remaining alive, and vaquitas, which are down to 10 individuals.
While de-extinction technologies await their first mammoth birth, the tools developed for this moonshot have already transformed our food systems. Companies like Inari are deploying similar multiplex editing systems to create climate-resilient crops that reduce breeding timelines from decades to years. Genus has used these techniques to develop PRRS-resistant pigs that could save the pork industry billions of dollars annually. The reproductive technologies vital for de-extinction also enable Roslin Technologies to produce specialized animal cell lines for cultivated meat, while Pairwise’s CRISPR platform has already launched gene-edited mustard greens with reduced bitterness.
Beyond agriculture, these technologies have accelerated progress across biomedicine and industrial biotechnology. Fauna Bio’s platform, for example, mines data from 290+ animals to uncover naturally evolved protective mechanisms, like those preventing muscle atrophy in hibernating bears, that can be translated into human therapeutics. Another company, eGenesis, co-founded by George Church (also of Colossal Biosciences), applies the same multiplex editing techniques to develop humanized pig organs for transplantation, gaining FDA clearance for clinical trials of gene-edited pig livers in April 2025. Ginkgo Bioworks extends these synthetic biology tools to industrial applications, engineering microbes that produce everything from fragrances to pharmaceuticals.
The convergence of these technologies marks a historic inflection point. While researchers debate whether Colossal Biosciences' approach to hybrid animals constitutes true de-extinction, the tools being used have given humanity unprecedented power to rewrite Earth's biological operating system. From reviving lost ecosystem functions to engineering disease-resistant crops and reimagining industrial production, the science behind second chances is transforming how we interact with life itself and reshaping our biological future at a planetary scale.