Actionable Summary
Signed in 2015, the Paris Agreement combines efforts from 196 countries to prevent the average global temperature from rising 2°C above pre-Industrial Revolution temperatures by 2050.
While the Paris Agreement is influencing much of the policy and funding around climate change, current research indicates that the world is already halfway towards passing this limit by 2040.
There are two possible methods of slowing down climate change: (1) reduce the amount of greenhouse gasses (GHGs) entering the atmosphere, or (2) remove the greenhouse gasses from the atmosphere via carbon removal.
Increasing the use of renewable energy is one path to preventing emissions. Renewable energy sources were first introduced in the 1880s through hydropower and are now growing at an increasing rate. The most popular forms of renewables (in order of energy production) are nuclear, hydroelectric, wind, and solar. Others include biomass, geothermal, wave, etc.
Carbon removal can take many forms, but the most common are (1) carbon sequestration: sequestering the emissions at the point of production and (2) direct air capture (DAC), which uses large fans to suck carbon out of the air.
Carbon credits, tokens which each represent one ton of carbon removed from the environment, have become a common way for people and businesses to offset their emissions. While businesses should decarbonize their operations (i.e. switch to renewable energy), certain emissions are not removable given current technology. By purchasing offsets for any remaining emissions, businesses can claim they are “carbon neutral”.
Many startups are emerging in an attempt to bridge the gap in creating the technology necessary to limit the use of fossil fuels. These startups fall into four main buckets: energy related, science related, climate adaption, and carbon accounting & offsets.
The Climate Conundrum
When it comes to climate change, consensus has it that the Earth is getting warmer and fossil fuels are a contributor. At the same time, fossil fuels are likely to remain in use for the foreseeable future. ~94% of all cars still ran on internal combustion engines as of 2022, and while that’s lower than the previous year at 97%, EVs remain a minority of vehicles globally. Further, fossil fuels are necessary for air travel and used in a wide array of everyday items – paint, plastics, asphalt, and more.
It’s common to address climate-related topics by starting with the nuances of a particular category, whether that's carbon accounting, carbon sequestration, or some other specific component. The focus of this report, by contrast, is on unpacking the path towards decarbonization as an ecosystem of solutions that are interconnected: from how the climate discussion has evolved, to what solutions have emerged to date, to the most likely trends that will make a difference in addressing climate change before it becomes unaddressable.
The Climate Change Discussion
The Paris Agreement was signed by 196 countries on December 12, 2015 and put into effect on November 4, 2016. Its overall goal was to coordinate a global effort to limit the increase in Earth’s average temperature to less than 2 degrees Celsius (°C) above pre-industrial levels. Each country agreed to work together to limit the increase in global temperatures to less than 1.5 degrees by the end of the century.
But what are “pre-industrial levels?” In 2022, the average global temperature was 58.6 °F, about 1.9 °F higher than the mid-late 1800s, which is generally considered the tail end of the Industrial Revolution (1760-1840). According to an article published by NASA in 2017:
“The planet’s average surface temperature has risen about 2.0 °F (1.1 °C) since the late 19th century, a change driven largely by increased carbon dioxide and other human-made emissions into the atmosphere. Most of the warming occurred in the past 35 years, with 16 of the 17 warmest years on record occurring since 2001.”
So a reasonable estimate of the pre-industrial average temperature seems to be around 56.7 °F. The Paris Agreement’s goal is to keep temperatures under 59.4 °F. An IPCC prediction showed this limit was on track to be exceeded by 2040, which was a big driver behind the commitments so many countries made in signing the Paris Agreement.
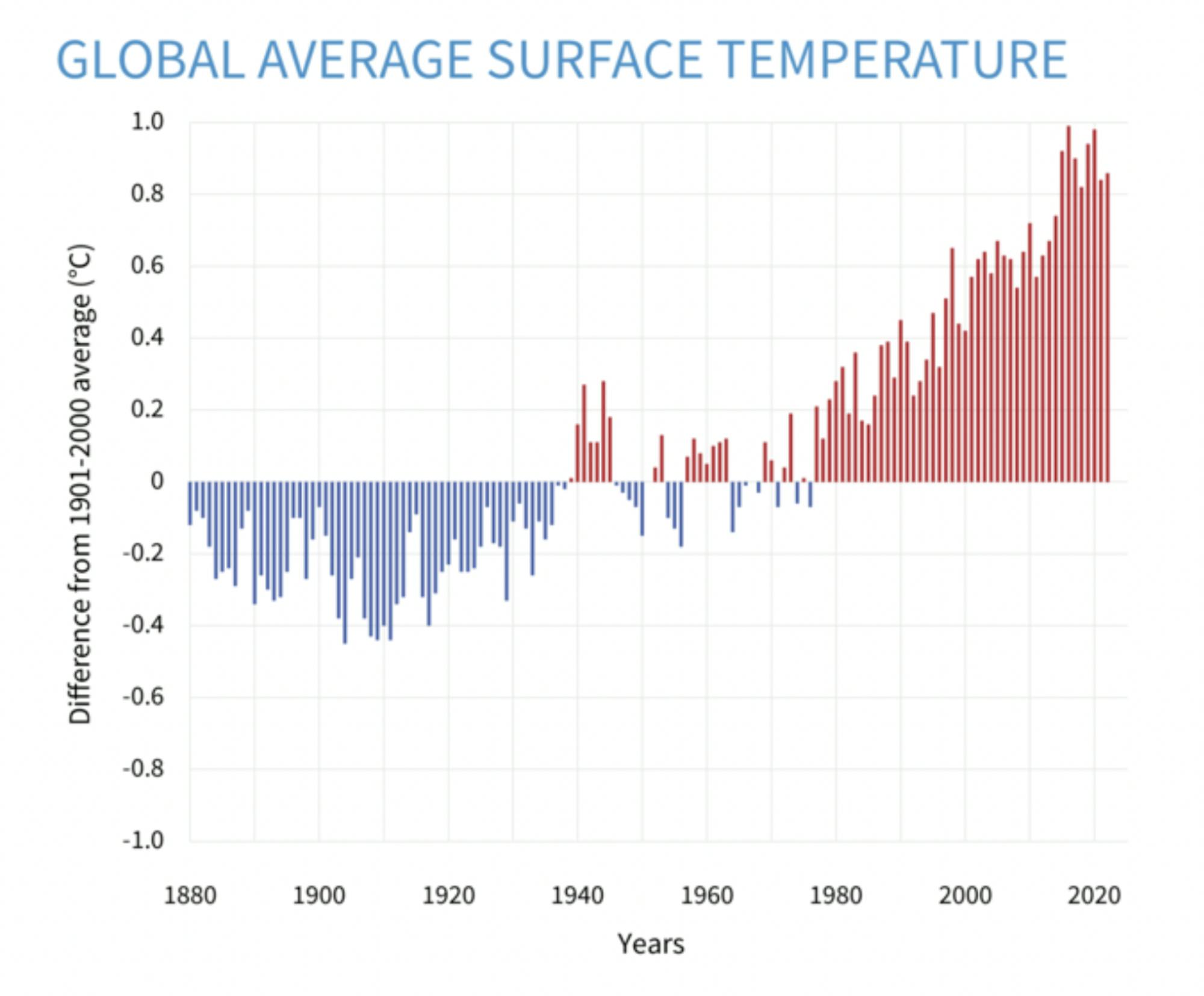
Source: NOAA
The invention of the steam engine kicked off the industrial revolution and enabled fossil fuels, starting with coal, to be converted into usable energy. A byproduct of this was an increase in the emission of carbon dioxide, which grew rapidly in the late 20th and early 21st century as much of the globe industrialized.
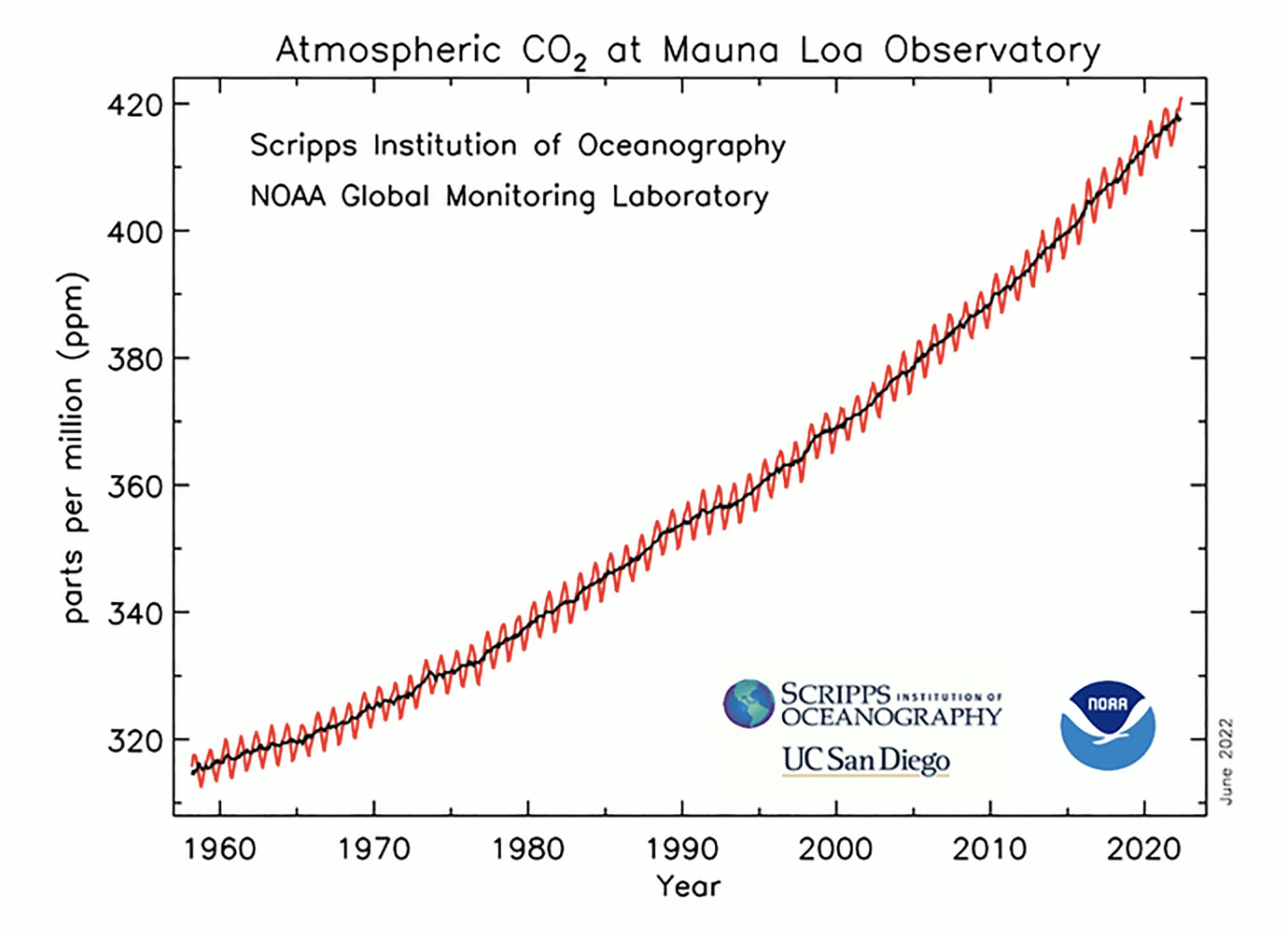
Source: Global Monitoring Lab
Carbon dioxide, however, is not the only molecule that worries climate scientists. Methane and nitrous oxide are the two largest sources of GHG emissions behind carbon dioxide, and each have their associated pros and cons. While methane is short lived compared to carbon dioxide, thus making it less of a long-term problem, it is 25x more potent when released to the atmosphere, making it extremely hazardous in the short-term. Similarly, nitrous oxide is short-lived but 300x more potent than carbon dioxide in terms of its impact on the climate.
Unprecedented Human Impact
So carbon emissions have gone up considerably since the Industrial Revolution and now everyone wants to stop the rise in global temperature by the end of the century. But what is the most common counterargument to people’s concerns over rising global temperatures? It goes something like this – the Earth has been warming and cooling in cycles over millenia for any number of reasons. From at least the five major ice ages that have occurred in Earth’s past, to 115K years ago when the Earth was as hot as it is now, the range in global temperatures has been fairly large over the course of life’s long history on Earth.
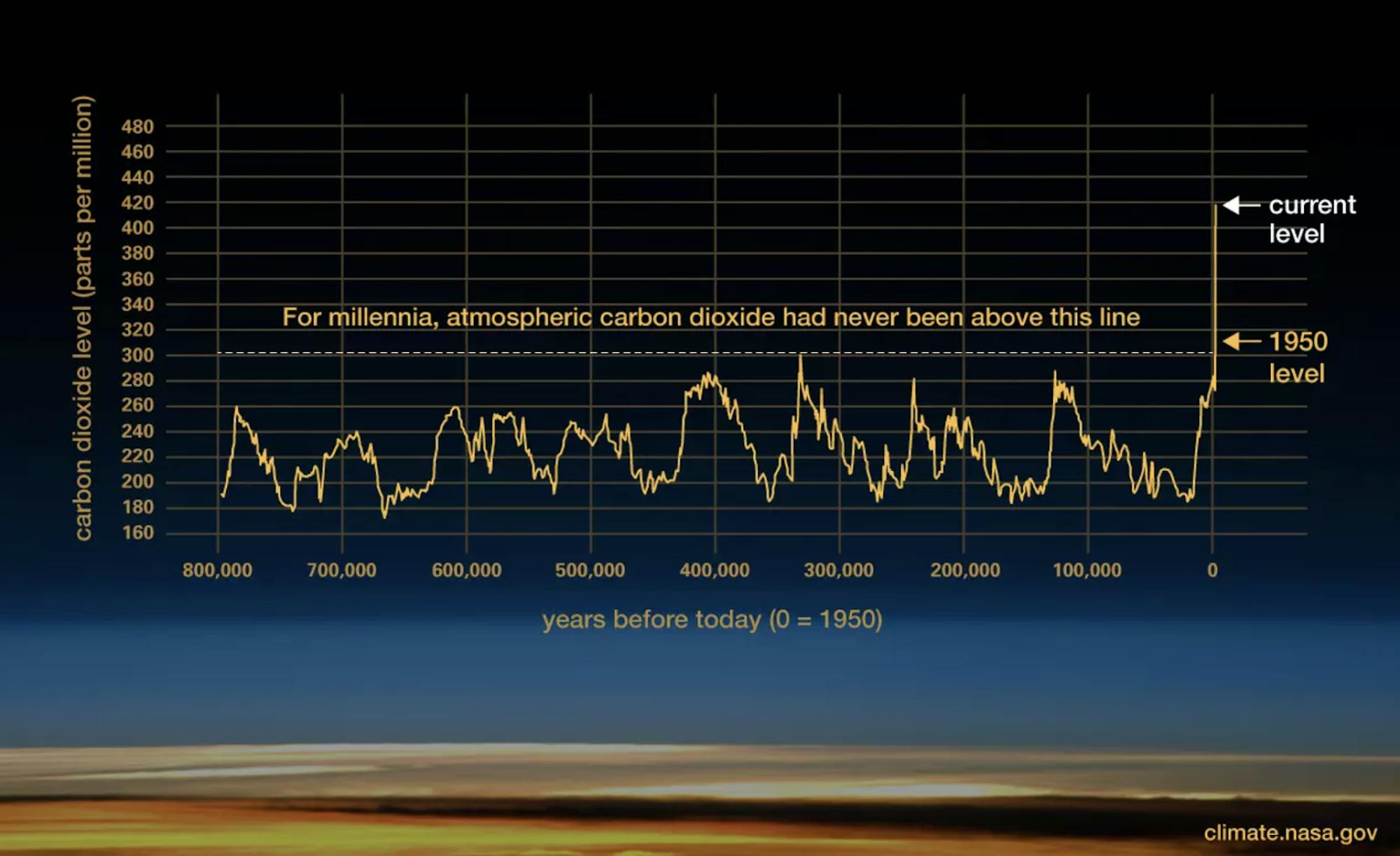
Source: NASA
However, while it’s true that the Earth has been this warm before, there is no precedent for the rate of growth in carbon dioxide levels that we are now seeing. It’s the rate of change that is the problem. As NASA puts it:
“As the Earth moved out of ice ages over the past million years, the global temperature rose a total of 4 to 7 °C over about 5,000 years. In the past century alone, the temperature has climbed 0.7 °C, roughly ten times faster than the average rate of ice-age-recovery warming.”
Carbon levels are rising because fossil fuel is burned to produce and power almost everything in the world. Bill Gates’ book How to Avoid a Climate Disaster observes that humans treat fossil fuels like water. Everyday use of things like plastic (toothbrushes, bottles, bags) implies the use of fossil fuels, since plastics are made with petroleum. A majority of food in many countries is grown with GHG-emitting fertilizers and is harvested by gas-powered tractors made of steel, which itself requires fossil fuels to produce. Red meat, on top of this, comes from animals that produce a lot of methane. Such goods are also shipped to markets for retail over land, sea, and air using gas engines. Much of the modern economy, from the production of food and clothing to construction and transport, has steps involving the consumption of fossil fuels and the emission of greenhouse gasses.
Fossil fuels are aptly named – they’re simply fossils – mostly made up of plant matter from millions of years ago. When these fossilized plants (oil/gas/coal) are burned, they release organic compounds, namely carbon. So there is a direct link between increases in carbon and fossilized carbon matter being burned.
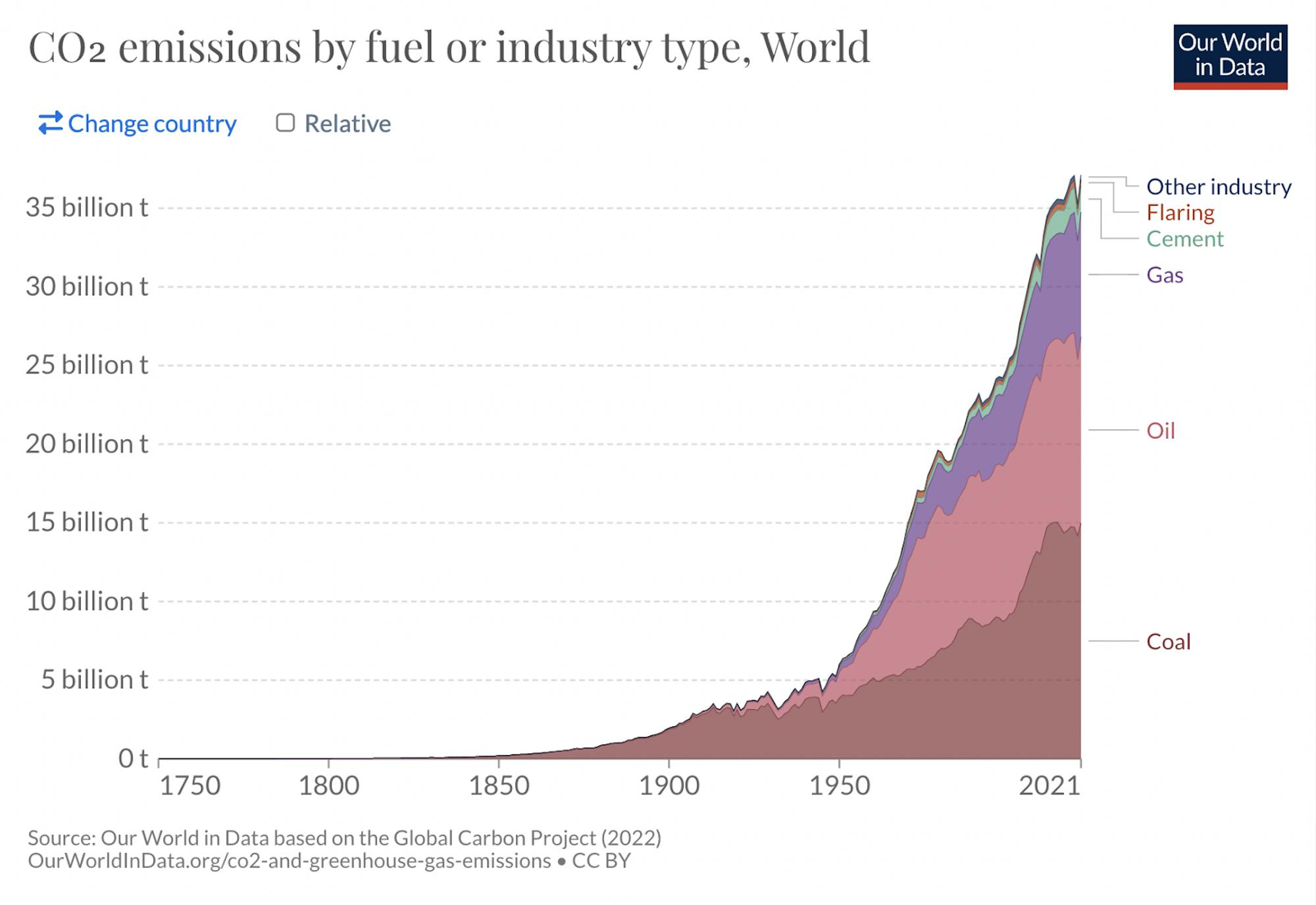
Source: Our World in Data
Notice that coal made up 42% of carbon dioxide emissions in 2021 but it actually only accounted for about 27% of the world’s energy supply. This is a stark example of just how much carbon dioxide fossil fuels emit. As the EPA put it in 2014:
“Global carbon emissions from fossil fuels have significantly increased since 1900. Since 1970, carbon dioxide emissions have increased by about 90%, with emissions from fossil fuel combustion and industrial processes contributing about 78% of the total greenhouse gas emissions increase from 1970 to 2011. Agriculture, deforestation, and other land-use changes have been the second-largest contributors.”
Additionally some of the hottest years on record have occurred after 2011. At current rates, temperatures will likely reach 2.7 °F above pre-industrial levels by 2050 and between 3.6-7.2 °F by 2100.
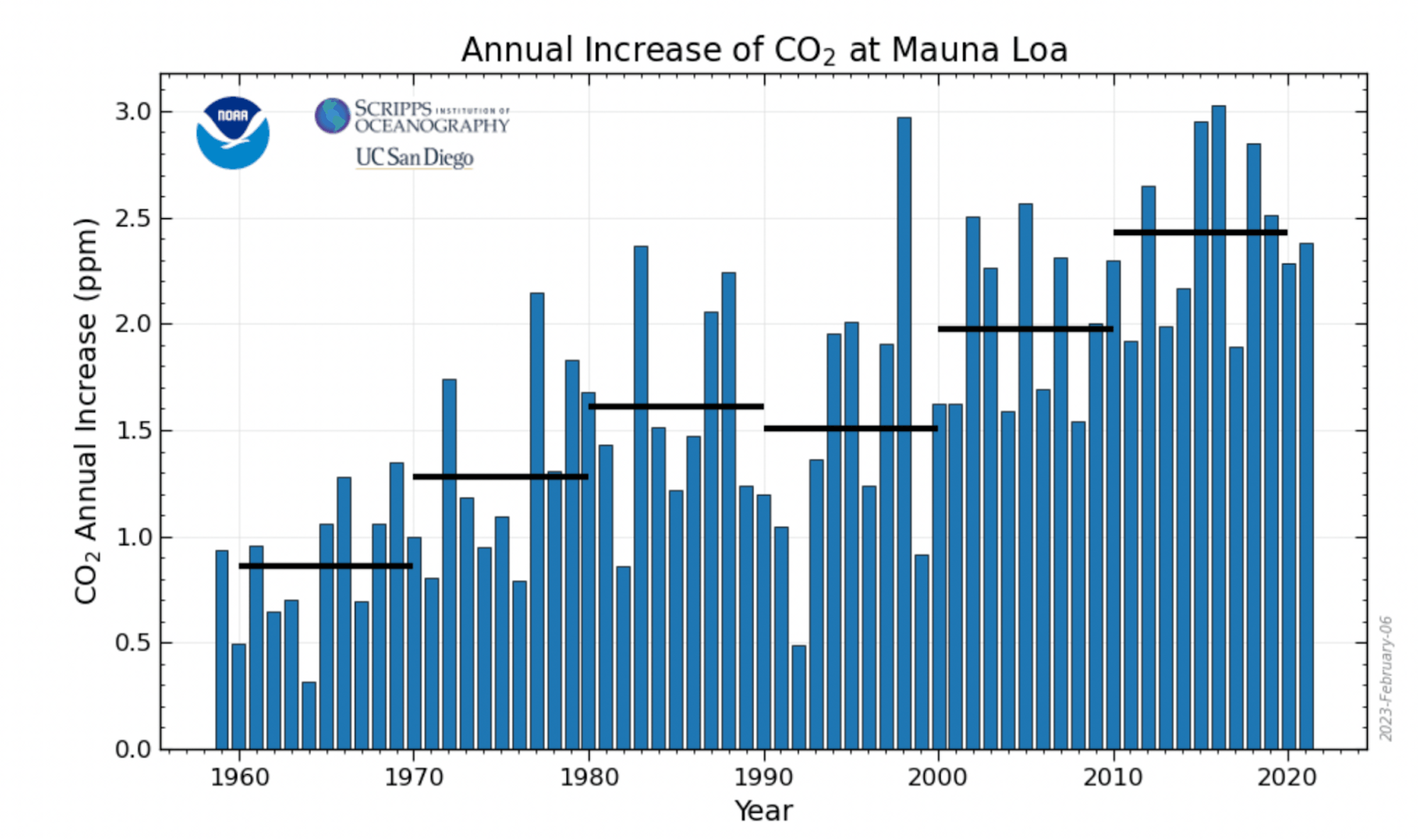
Source: Global Monitoring Lab
This implies that something has to change to meet the goal of the Paris Agreement. While scientists can speculate on what would happen to the Earth based on certain average temperatures, it is estimated that sea levels will rise, heat waves will become more common, and natural disasters could be more fierce.
One study estimated that the frequency of a major heat wave would increase by 92% at above 4° C pre-industrial levels. In addition, the melting of the polar ice caps means that more water has been activated into the weather system and therefore, when that water evaporates, more water is held in the clouds and therefore, there is more rainfall.
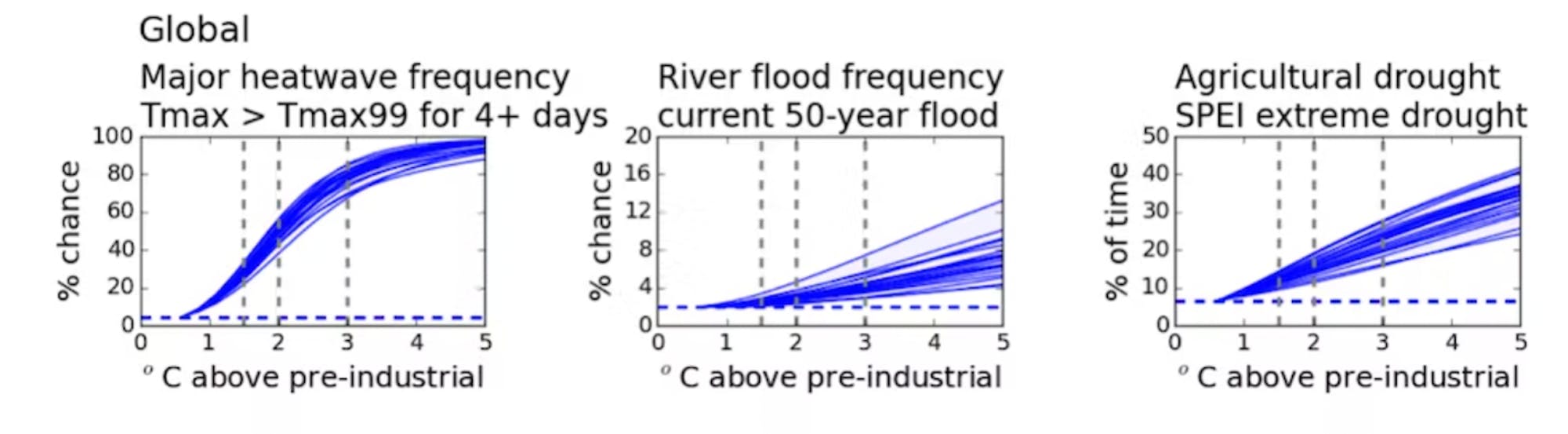
Source: Springer Link
The most recent affirmation of the Paris Agreements was in Glasgow in 2021, where policymakers recognized that in order to limit the increases to only 1.5 °C, greenhouse gasses would need to be cut by 45% compared to 2010 levels and get to carbon neutrality by 2050. Importantly, the US has pledged to get to carbon neutral by 2050, China by 2060 and India by 2070. With two of the world’s biggest economies not pledging to be carbon neutral by 2050, it’ll be difficult to limit the increase to 1.5 °C.
But it’s not just the fact that the atmosphere is heating up that creates a ticking clock situation. Another issue is that fossil fuels aren’t renewable sources of energy. One Stanford study from 2019 estimated that oil reserves would run out by 2052, natural gas by 2060 and coal by 2090. One thing these estimates don’t account for is the supplemental energy supply that can come from renewables in the meantime. So what approaches to renewable energy have people tried historically to tackle this problem?
History of Renewable Energy
In 2022, fossil fuels still accounted for roughly 80% of the production of global energy. Solar, wind, hydro, and nuclear were responsible for the majority of the remaining production.
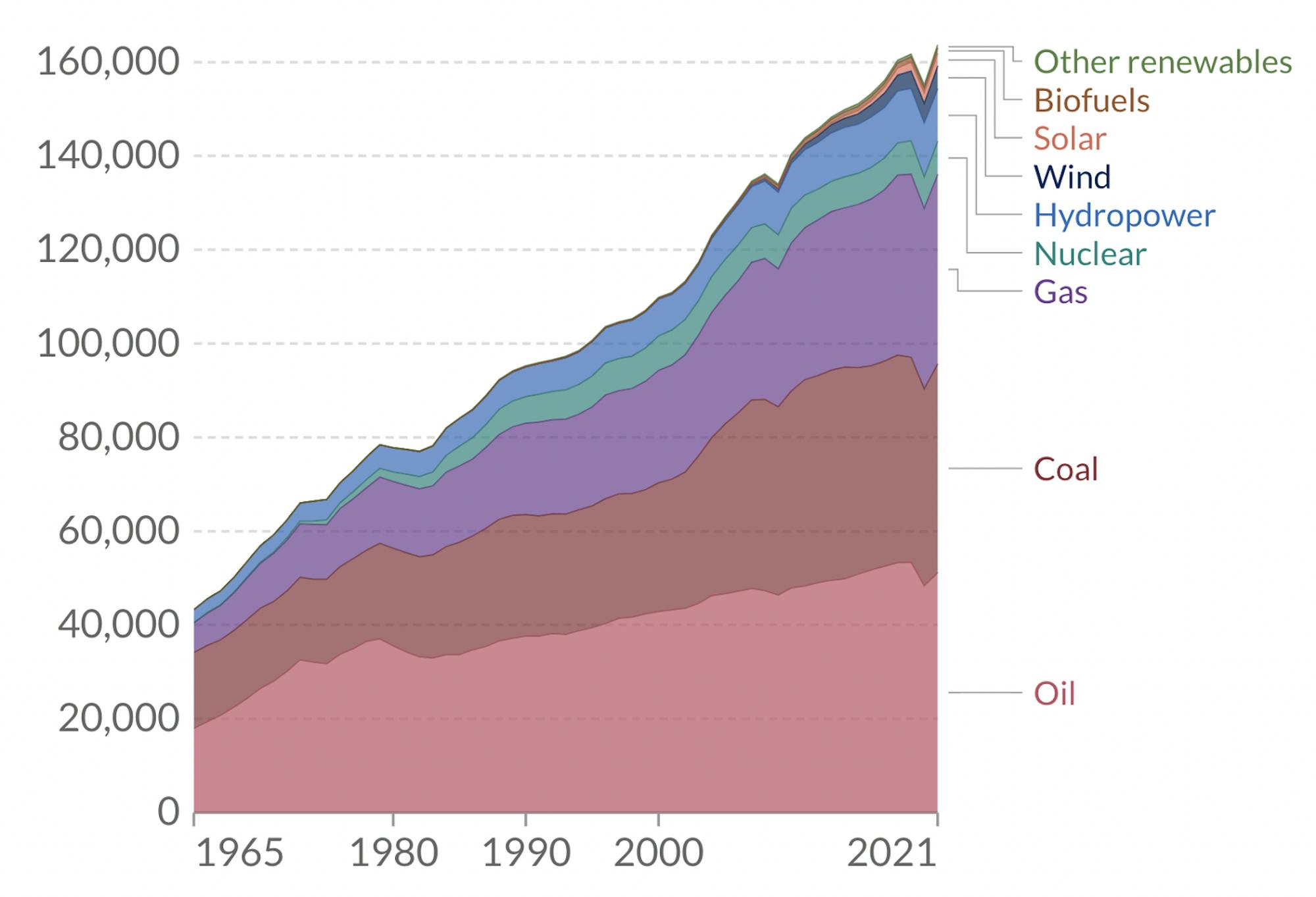
Source: Our World in Data
The first recorded instances of renewable energy being used by a society include boat propulsion across the Nile by 5,000 BC and windmills used to pump water in China by 200 BC, but the first use of renewables to create electricity didn’t happen until 1882. In 1882, the Vulcan Street Plant on Fox River in Appleton, Wisconsin became operational. Following this, 50 hydroelectric generating projects were announced across North America over the next four years. Hydro power quickly became the most popular source of renewable energy, and continues to account for most of the clean energy produced in the US.
Shortly after, wind power came to the US from Europe in 1888. Though solar power was being researched around the same time, the use of silicon photovoltaic (PV) cells to create electricity for everyday equipment didn’t occur until 1954. In the 1960s, Americans began to take notice of pollution due to disasters such as oil spills off the shore of California, chemical contamination of the Cuyahoga River (which spontaneously combusted), and city air quality deterioration. Around the same time, the US had just put a person on the moon, and the first photographs showing the entire Earth were taken. As a result, more people began to appreciate the fact that the Earth’s resources are finite.
Changing sentiment led President Nixon to present a message on the environment in 1970, requesting funding for water treatment facilities, national air quality standards, and research to reduce automobile pollution. As a result, he created the Environmental Protection Agency (EPA) to consolidate federal government programs to efficiently address environmental goals. The EPA would have the capacity to research pollutants and their impact on the environment, monitor the condition of the environment, establish environmental baselines, and set and enforce standards for air and water quality.
Reducing global emissions became a focus of the late 20th century. That period saw the first World Climate Conference in 1979, and other events like the United Nations Conference on Environment and Development (UNCED) in 1992. But in the early 2000s, the movement began to be more codified with the creation of ESG.
Birth of ESG & Carbon Credits
ESG, short for environmental, social, and governance, was an acronym first coined in the 2004 report "Who Cares Wins", a joint initiative of financial institutions in response to the United Nations' call for corporate social responsibility. Since then, ESG has evolved into a global movement, with supporters representing over $80 trillion in assets under management. The US government and other countries have used ESG practices and guidelines to inform decisions on capital allocation across capital intensive projects such as direct air capture and others.
Despite the increased popularity of ESG, some critics argue that ESG-linked products have not had, and are unlikely to have, the intended impact of raising the cost of capital for polluting firms. Critics have also accused ESG proponents of greenwashing, a marketing tactic used to promote a false image of environmental responsibility. For example, EV manufacturer Tesla has been given a lower ESG rating than multiple gas companies.
However, proponents of ESG argue that it provides investors with a more holistic view of a company's potential climate impact. Understanding a company’s global risks and opportunities leads to better-informed investment decisions. The debate around ESG’s impact on capital allocation and decision making remains hotly contested. However, one definitive effect of ESG investing has been the emergence of carbon credits and carbon markets.
Carbon credits are permits that allow companies to emit a certain amount of greenhouse gasses. Each carbon credit represents one ton of carbon emissions prevented from entering the atmosphere whether by carbon emissions prevention, avoidance (use of renewable energy), or some form of carbon sequestration. Carbon markets enable companies to buy or sell these permits, which creates an economic incentive for firms to reduce their carbon emissions.
Companies may choose to voluntarily purchase credits to reduce their emissions because of their sustainability values, or they may choose to purchase credits due to government emissions regulations. While organizations are first encouraged to reduce emissions by transitioning to clean energy, improving logistics, etc, the remaining emissions which cannot be reduced are covered by offsets.
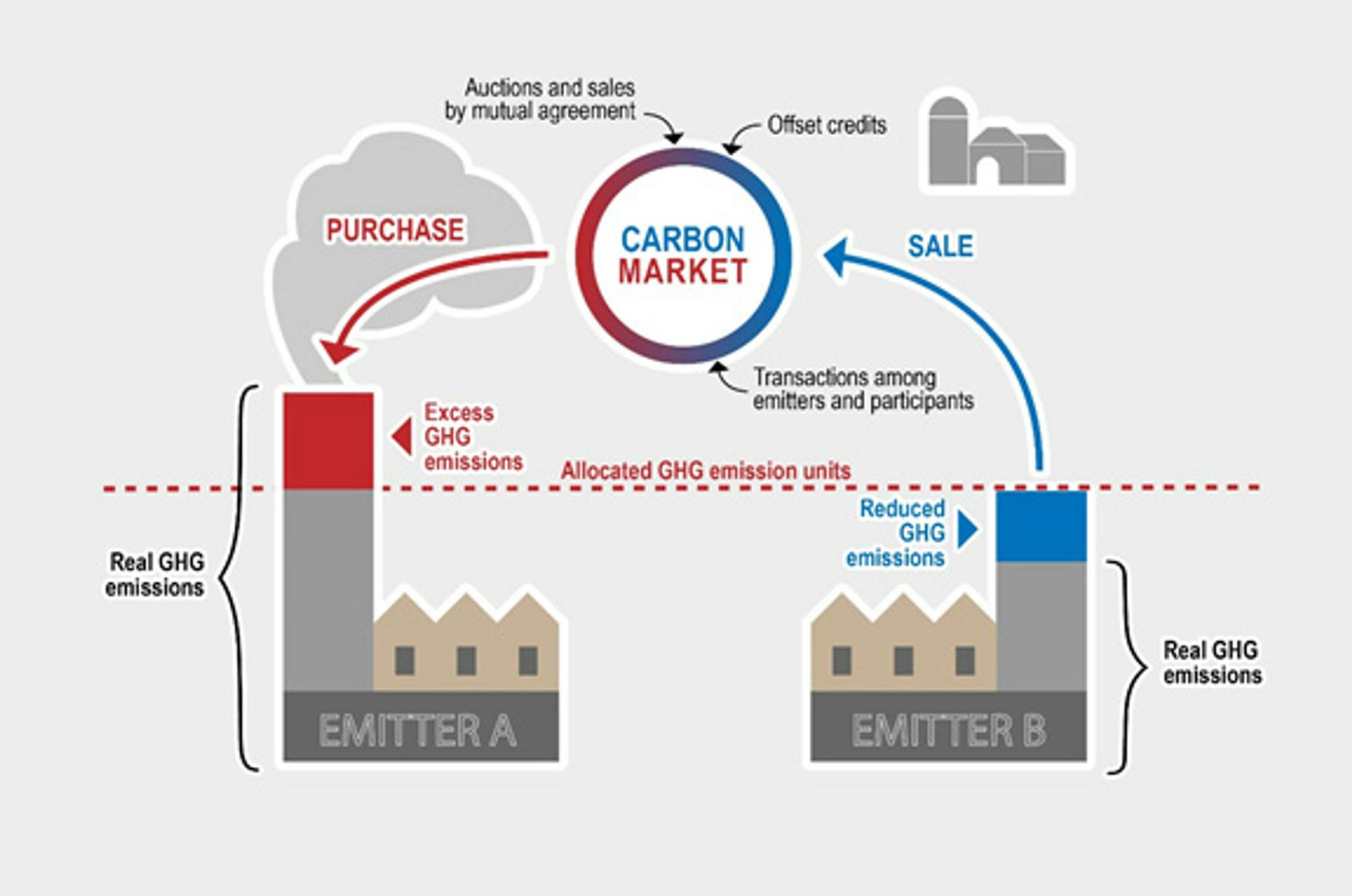
Source: CarbonRX
Carbon markets were popularized in 1997 when the Kyoto Project established the first international carbon market, which was then followed by the European Union Emissions Trading System (EU ETS) in 2005. Today, carbon markets exist in various forms across the world. The largest emissions trading system (ETS) is in China, followed by others including markets in the European Union, South Korea, and the US.
Money transacted in carbon markets is used as funding for the projects which sell the credits. The largest category for fund allocation is currently renewable energy projects, such as wind and solar power, but funds are also pushed into improvements in energy efficiency, carbon capture, and land use for reforestation.
Carbon markets have experienced significant growth over the past decade, with the global carbon market value having approached $1 trillion in 2023. This growth is expected to continue as more countries participating in the Paris Agreement and other emissions treaties enforce stricter policies on companies. Some estimates indicate demand for carbon credits could see exponential growth if adherence to the Paris Agreement remains strict.
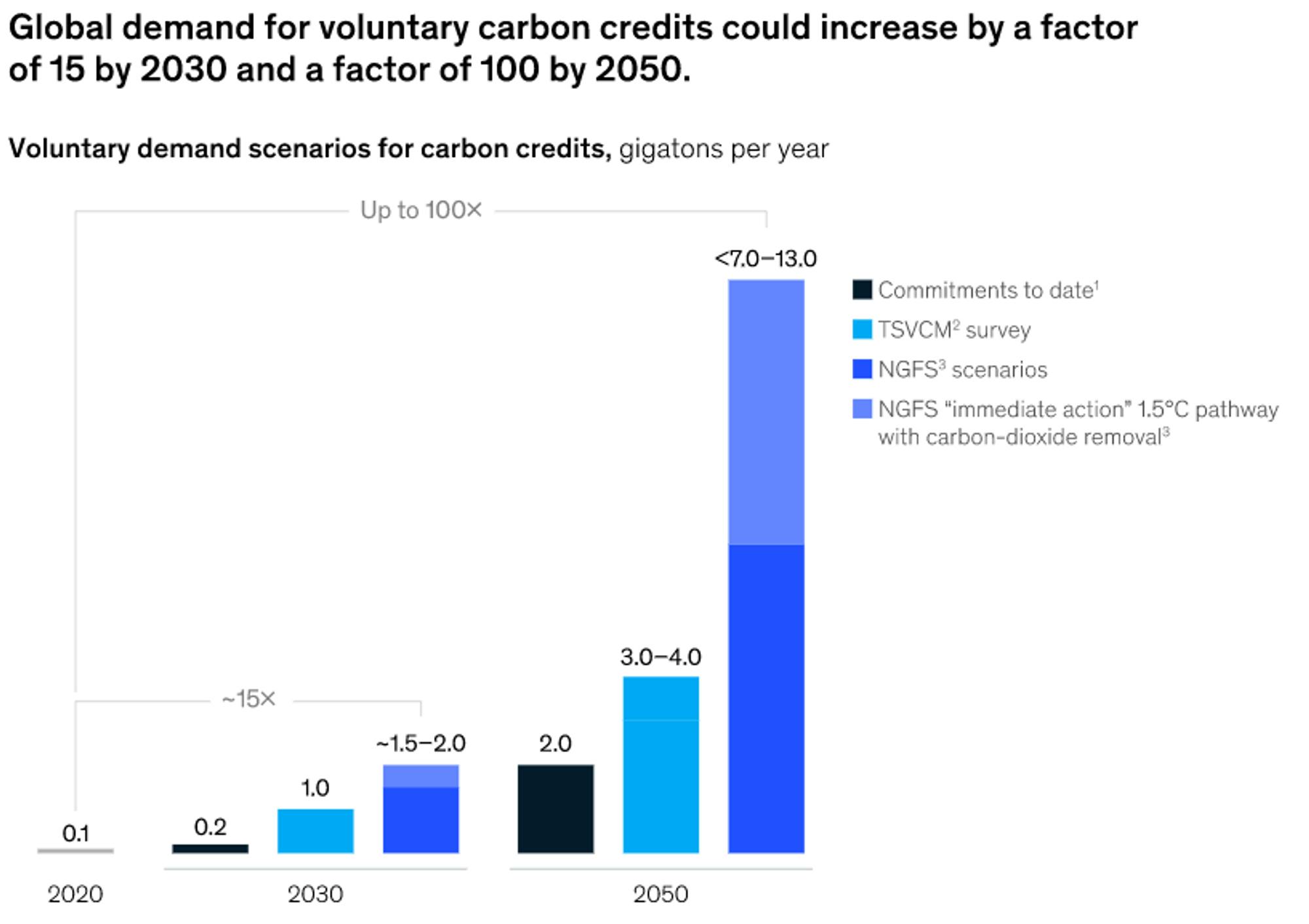
Source: McKinsey
15% of global emissions in 2020 came from extracting and transporting fossil fuels themselves, let alone the emissions from burning those fossil fuels. To meet emission reduction targets, the oil and gas sector must prioritize cost-effective interventions such as process changes and adjustments to reduce energy consumption. Many companies have already adopted techniques such as improved maintenance routines, vapor-recovery units, shifts to clean energy for extraction, use of vegetable oils as feedstock, and more. One option is to implement initiatives that offset emissions by tapping into natural carbon sinks, including oceans, plants, forests, and soil. Companies can invest in offsets, and upstream and downstream operators have different sets of options at their disposal.
In 2018, about 1% of gas and oil company budgets were spent on investment in green energy. However, that number has been increasing. For example, BP has invested hundreds of millions of dollars into solar power, EV charging stations, and wind power. Additionally, Shell planned to spend between $4 and $6 billion on green energy projects between 2016 and 2020. Chevron and ExxonMobil had also planned similar investments. These expenditures occurred in response to investor and consumer pressure, anticipated decline of fossil fuel consumption, the necessary diversification of business, and government incentives.
However, carbon markets are not without their critics. The key hesitation around carbon offsets is the reality that not all carbon offsets are created equal. There are three key characteristics that high-quality offset projects have in common:
Additionality: The GHG reduction being claimed wouldn’t have occurred without the purchase of an offset. For example, if a company purchases carbon offsets to allow landfill operators in California to install devices to capture and destroy methane, that does not qualify for additionality because, by law, landfill operators in California have to manage methane, so they would have done that with or without the purchase of an offset.
Avoided Overestimation: Some projects may struggle to accurately estimate the amount of carbon dioxide they’ve reduced. If a project is inaccurately reporting that they’ve reduced 100 tons, but in reality have only reduced 50 tons, then the value of that offset is overestimated.
Permanence: If an offset project is able to temporarily reduce some type of emissions, but then fails to maintain that reduction, then it fails to qualify as permanent. For example, if an emissions capture device on the smokestack of a manufacturing plant were to store carbon emissions, but the reservoir it was stored in had leaks, then the emissions are not being permanently reduced, just delayed.
Strict adherence to these three guidelines could lead to a significant blow to the perceived efficacy of carbon offsets. For example, avoided deforestation projects account for 90% of purchases offsets. But what if the forests were never in danger of being cut down? Or what if two companies account for not cutting down the same patch of land? Or what if they count it as an offset, but someone just cuts down the forest next year? In any of these cases, the project in question would not qualify as a high-quality offset.
New regulations have been put in place to try and standardize the quality of certain offset projects. But another obstacle there is that as of 2021, 60% of offsets that have been purchased were created in 2015 or earlier, when regulations were much less strict. Only 0.2% of offsets were created in 2021 or later when regulations became more strict. As a result, critics of carbon offsets see them as little more than an accounting trick to allow companies to claim carbon neutrality, while being provided with a blank check to continue emitting carbon. For example, Delta Airlines purchased offsets for 27 million tons in 2021. But an investigation of the underlying credits found that only ~6% were high quality offsets.
Skepticism around carbon offsets and the politicization of ESG have created reactions in the market. Many of the measures proposed by Chevron and ExxonMobil were struck down by shareholders in April 2023. At the same time, BP also rolled back several climate initiatives in favor of increased oil production driven by skyrocketing oil prices due, in part, to the conflict in Ukraine. Large asset managers, like BlackRock, who have pushed for climate-conscious measures among portfolio companies, faced backlash against ESG policies in 2023.
While carbon markets were intended to create incentives for companies to be more thoughtful of their carbon footprints, the increasing politicization of renewable energy and ESG efforts makes emission reduction and offsetting a much more difficult challenge. As a result, many climate activists have redirected their focus towards the second form of mitigating climate change: removing carbon directly from the atmosphere.
Carbon Capture
The process of removing carbon from the atmosphere can involve a number of different technologies and approaches. The primary umbrella is direct air carbon capture and sequestration (DACCS). Carbon sequestration is the process of storing carbon recaptured from the atmosphere. There are many forms of carbon sequestration, both naturally occurring and man-made. Carbon is sequestered everyday by forests, oceans, soil, grasslands and more which have naturally balanced the atmospheric levels of GHGs for millennia. Organisms like coral, seagrass, and other microorganisms found in the ocean remove substantially more carbon when compared to the same area of land.
There are also human-engineered ways of removing carbon from the atmosphere. First, carbon can be handled at the source of production then sequestered geologically by pumping it underground. For example, chemical manufacturing plants, which are responsible for nearly 30% of US GHG emissions, can store carbon dioxide instead of emitting it, and then these facilities can convert it into a liquid, and pump it underground into porous rocks for long term storage. This way, carbon dioxide never makes it into the air.
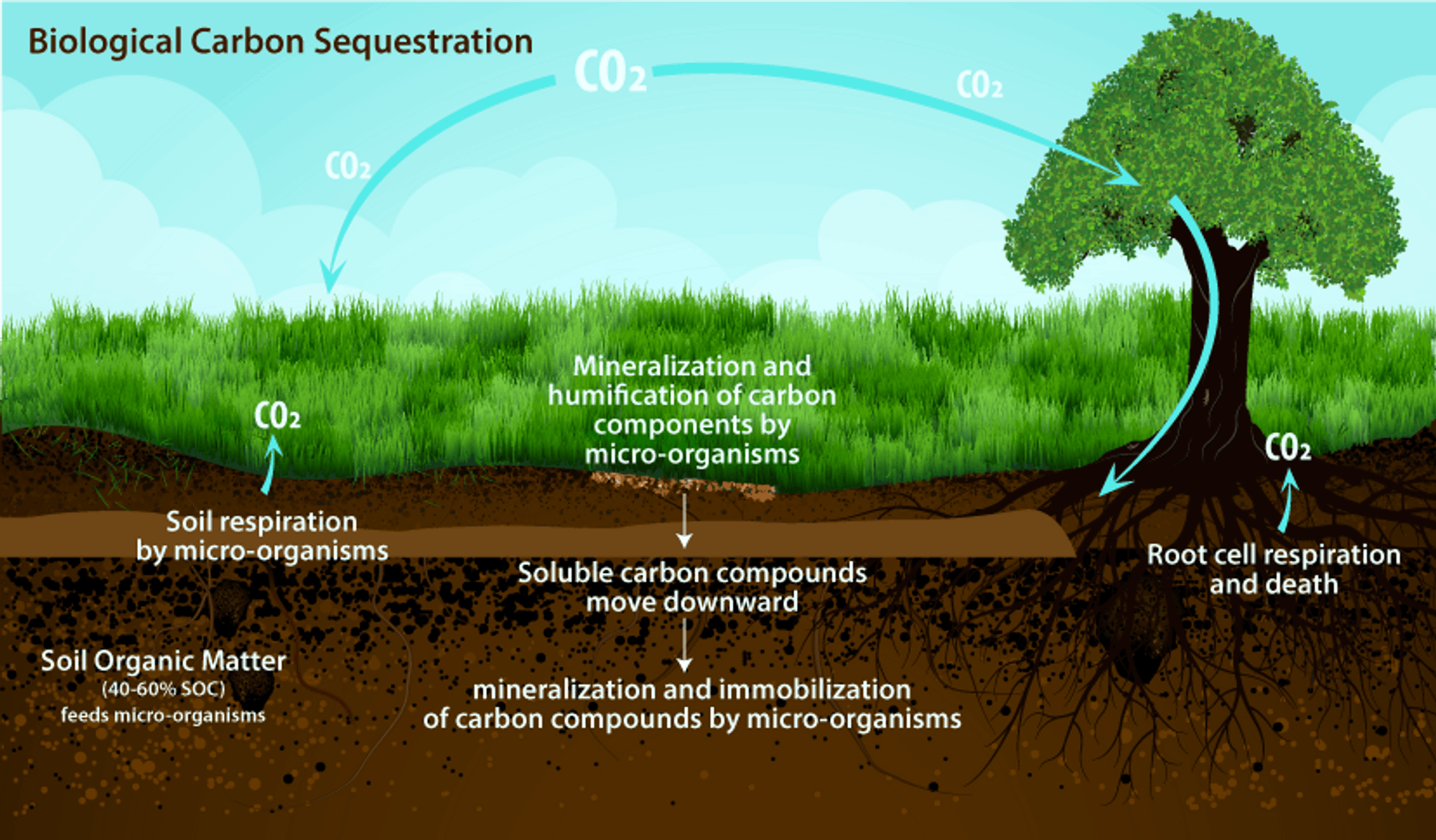
Source: CalRecycle
Direct air capture (DAC), the process of removing carbon dioxide which has already made its way into the atmosphere, uses industrial fans to pump large amounts of air through a system which identifies and filters carbon dioxide, then releases the carbon dioxide-reduced-air back into the environment. The idea for this was generated by a chemical engineer at Arizona State University in 1999 but did not become scalable until recently, with one the first large-scale DAC plant being commissioned in 2022.
There are two main approaches to DAC: Solid DAC (S-DAC) and Liquid DAC (L-DAC). S-DAC uses a solid adsorbent material to capture carbon dioxide from the air at low pressure and medium temperature. L-DAC uses a basic solution, like potassium hydroxide, to capture carbon dioxide from the air at high temperature. In both methods, once the carbon dioxide is captured, it can either be stored or used for other purposes, such as producing synthetic fuels.
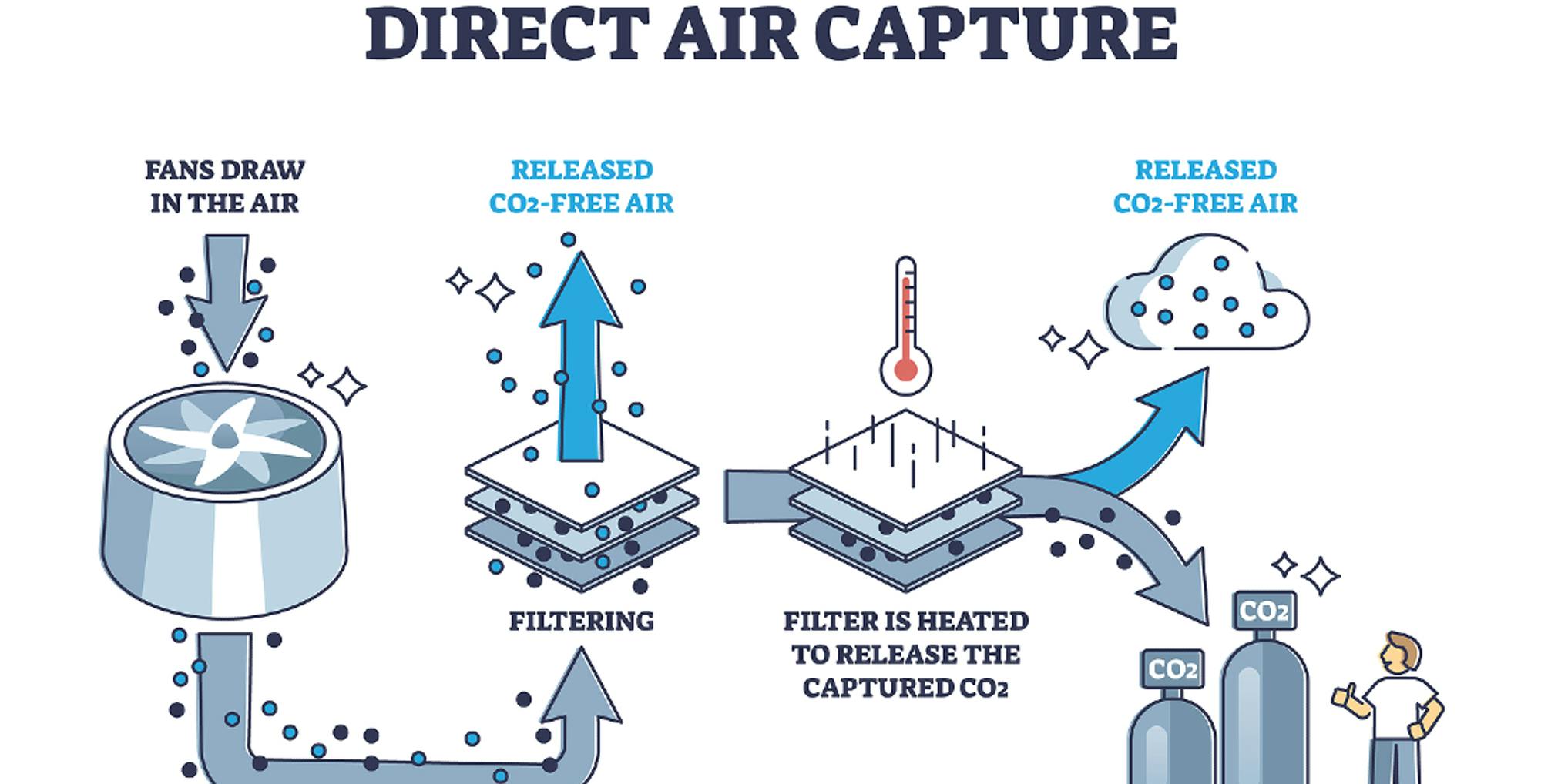
Source: Proximo
As of late 2022, there were 18 direct air capture plants in operation globally, which were capturing almost 0.01 metric tons (Mt) of carbon dioxide per year. A direct air capture plant in the United States that can capture 1 Mt of carbon dioxide per year is also in advanced development. In order to reach the goal of achieving net zero emissions by 2050, direct air capture needs to be scaled up to capture more than 10 giga tons (Gt) of carbon dioxide per year by 2050, eventually increasing to 20 Gt by 2100. To reach this lofty goal, several more large-scale demonstration plants are in development to fine-tune the technology and decrease capture costs. Currently, to remove one ton of carbon dioxide costs range from $500-$800 due to large infrastructure and energy requirements.
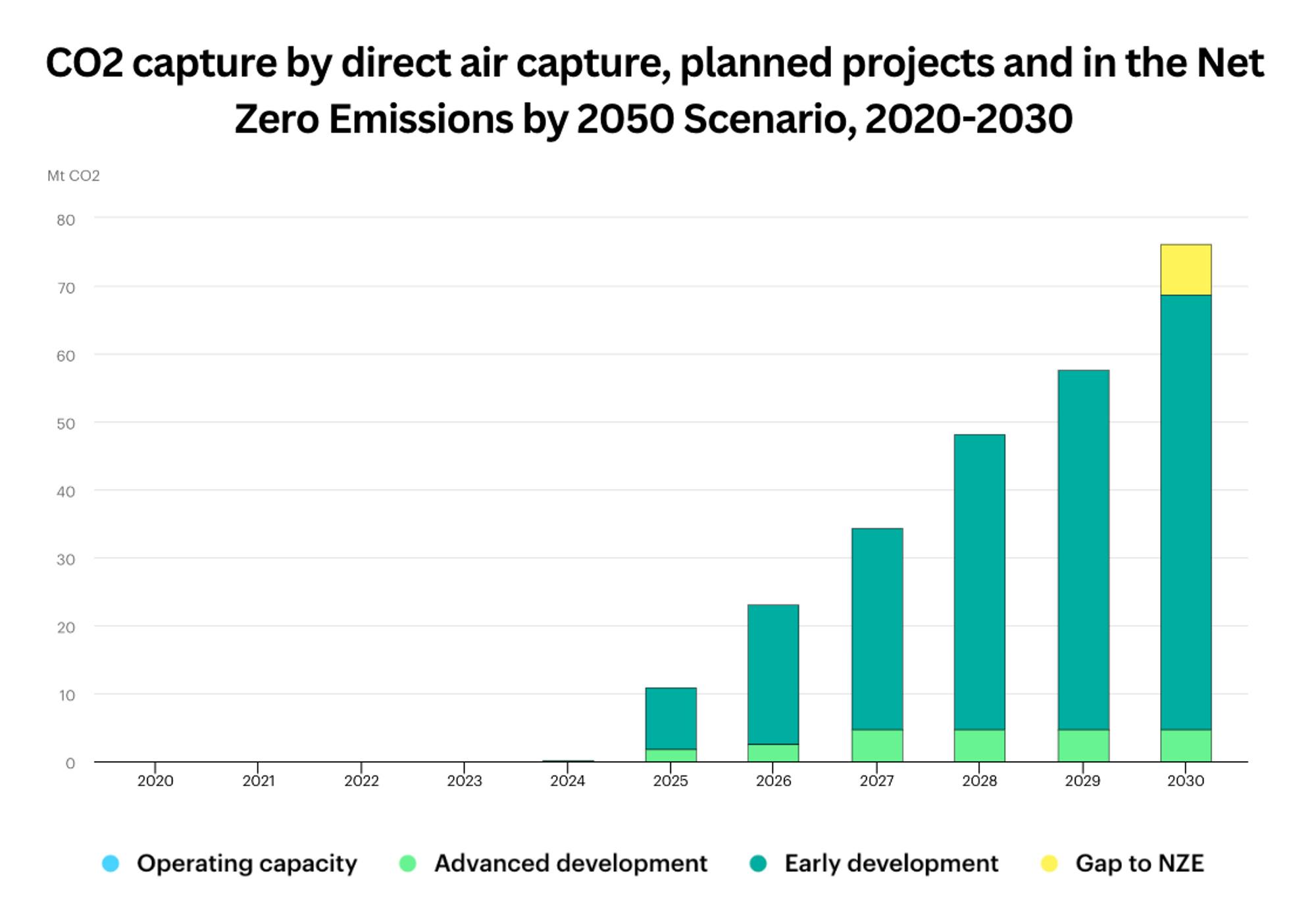
Source: IEA
For a deeper exploration of carbon removal, check out Industrializing Carbon Removal, an essay published in Foundations & Frontiers by Anna-Sofia Lesiv.
Proponents of goals like those set by the Paris Agreement are facing a combination of politicized emissions reduction efforts, technical and economic challenges with carbon removal, and the prospect of a looming event horizon beyond which the climate crisis becomes unfixable. Facing this dilemma, let’s unpack the current efforts being initiated to tackle these challenges.
Evaluating Various Renewable Energy Sources
Solar & Wind
One key advancement in the pursuit of a decarbonized world is the improving efficacy of solar and wind power. Renewable energy accounted for about 30% of the world's electricity generation in 2021. Solar and wind are the primary drivers of renewable energy growth since 2014, also representing the biggest source of additional electricity-generating capacity.
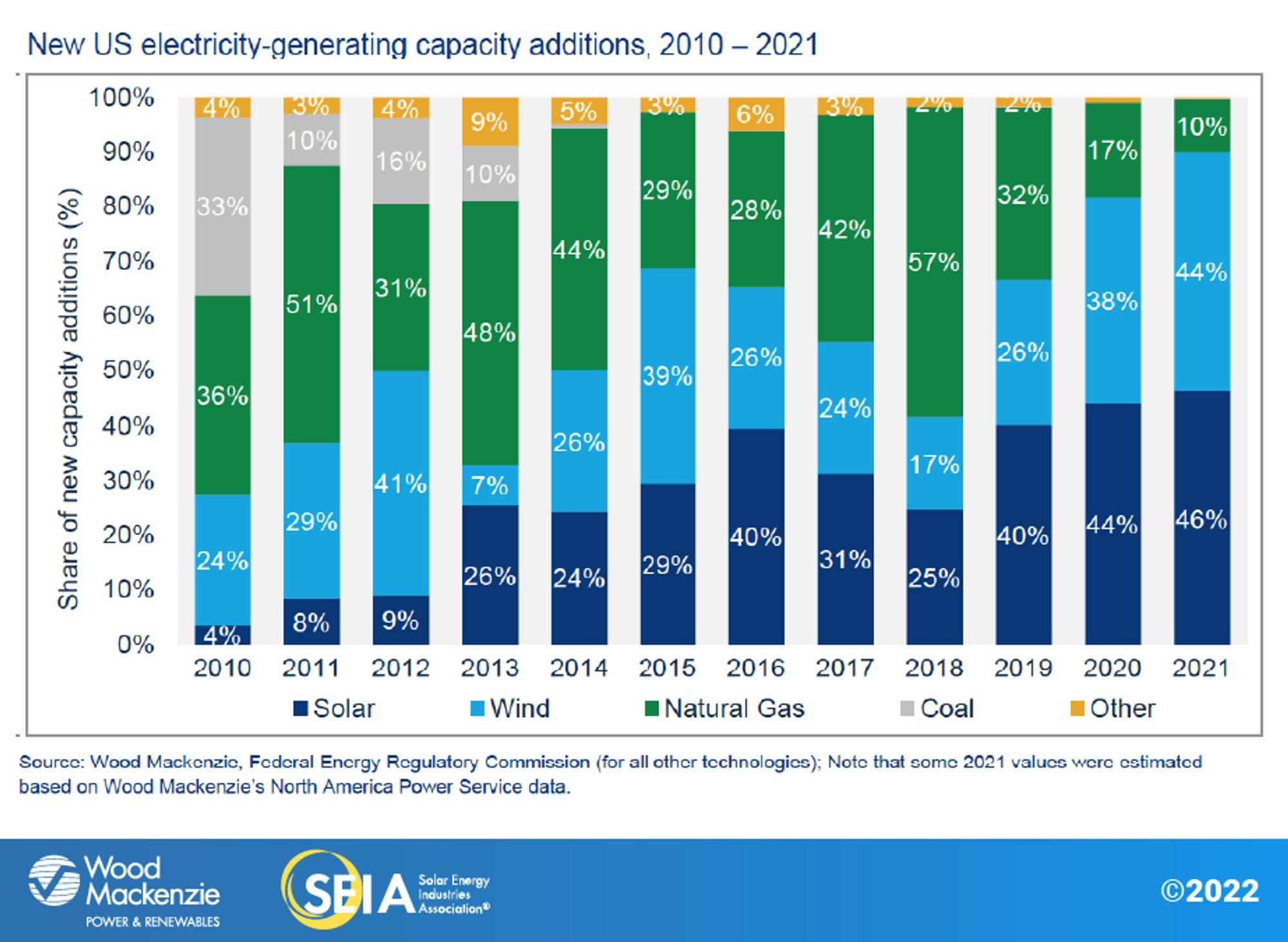
Source: SEIA
The market for solar power was growing at 20% YoY as of January 2023. The Inflation Reduction Act (IRA) that became effective in 2023 offers additional incentives for renewable energy adoption by lowering the costs of emission reduction and clean energy transitions. For example, tax incentives included in the IRA can offset the cost of residential solar installation by 30%.
Beyond incentives, the fundamental cost of manufacturing solar systems has also continued to come down. Between 2009 and 2019, the cost of a solar module dropped by 90% as countries like the US in the 1970s, Japan and Germany in the 1980s and 1990s, and China in the 2000s incentivized investments in renewable energy manufacturing.
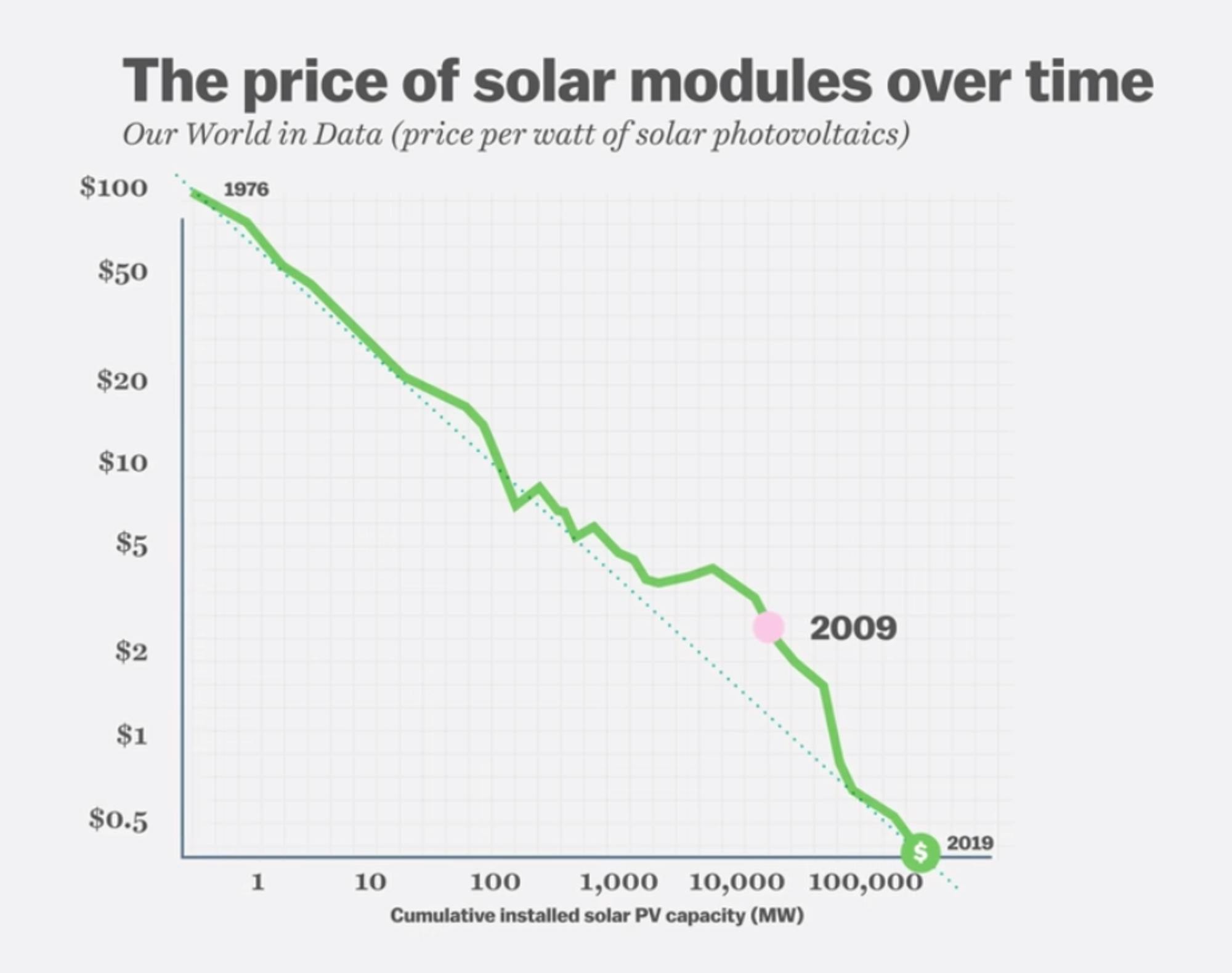
Source: Vox
As a result, the price to generate electricity via solar power has dropped below even natural gas by 2023. While this has been an exceptional shift in the cost of energy generation, that is only one part of the broader energy supply chain.
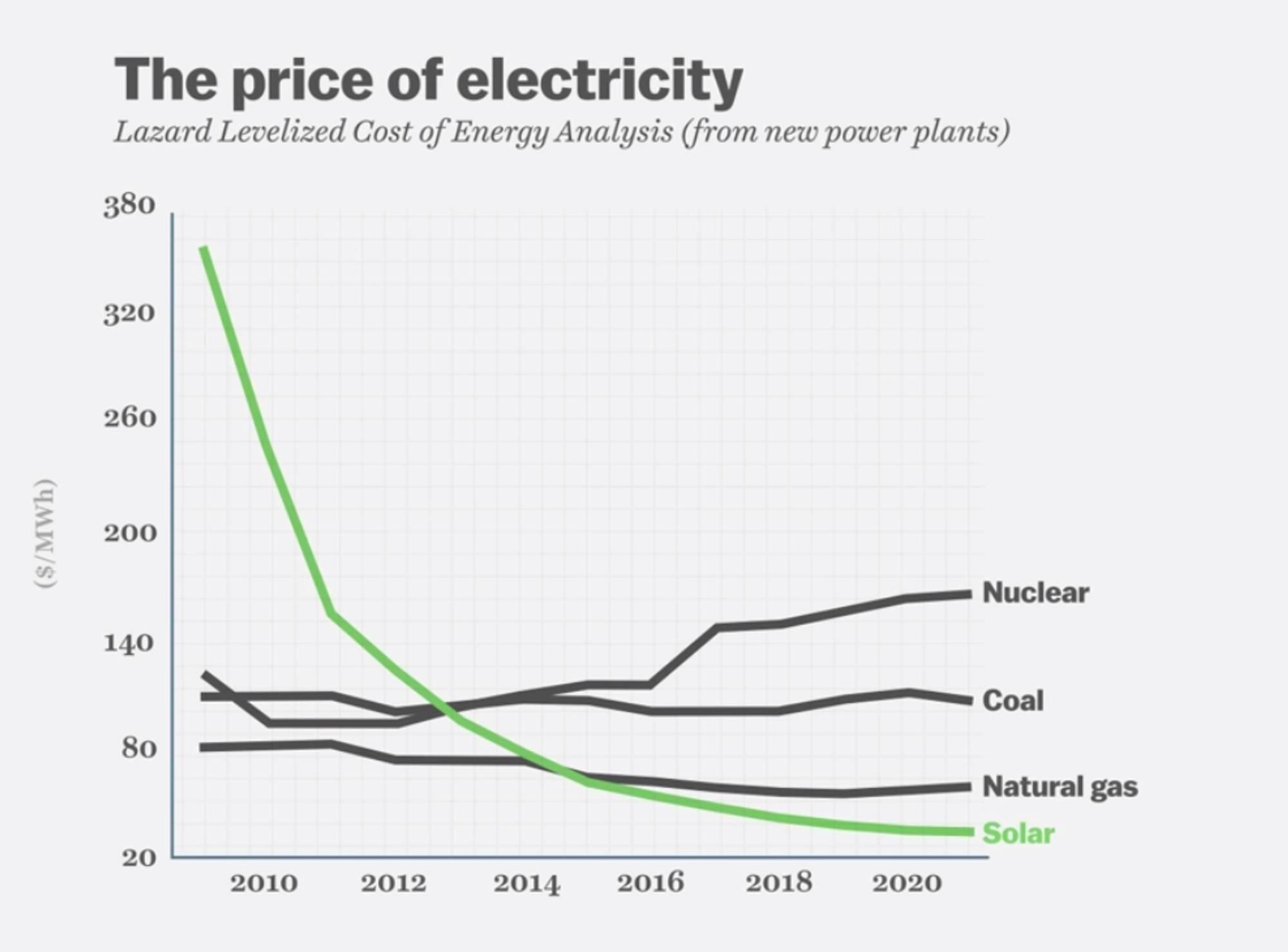
Source: Vox
Across the entire energy supply chain for solar power, 80% of the fundamental pieces are manufactured in China. Not only does this create an uncomfortable dependency in an increasingly deglobalized world, but many of the manufacturing facilities in China produce the materials using forced labor and other human rights violations.
In addition to continuing to improve the solar supply chain, there are other limitations for both solar and wind. Some of the key benefits and challenges of solar and wind power are summarized below.
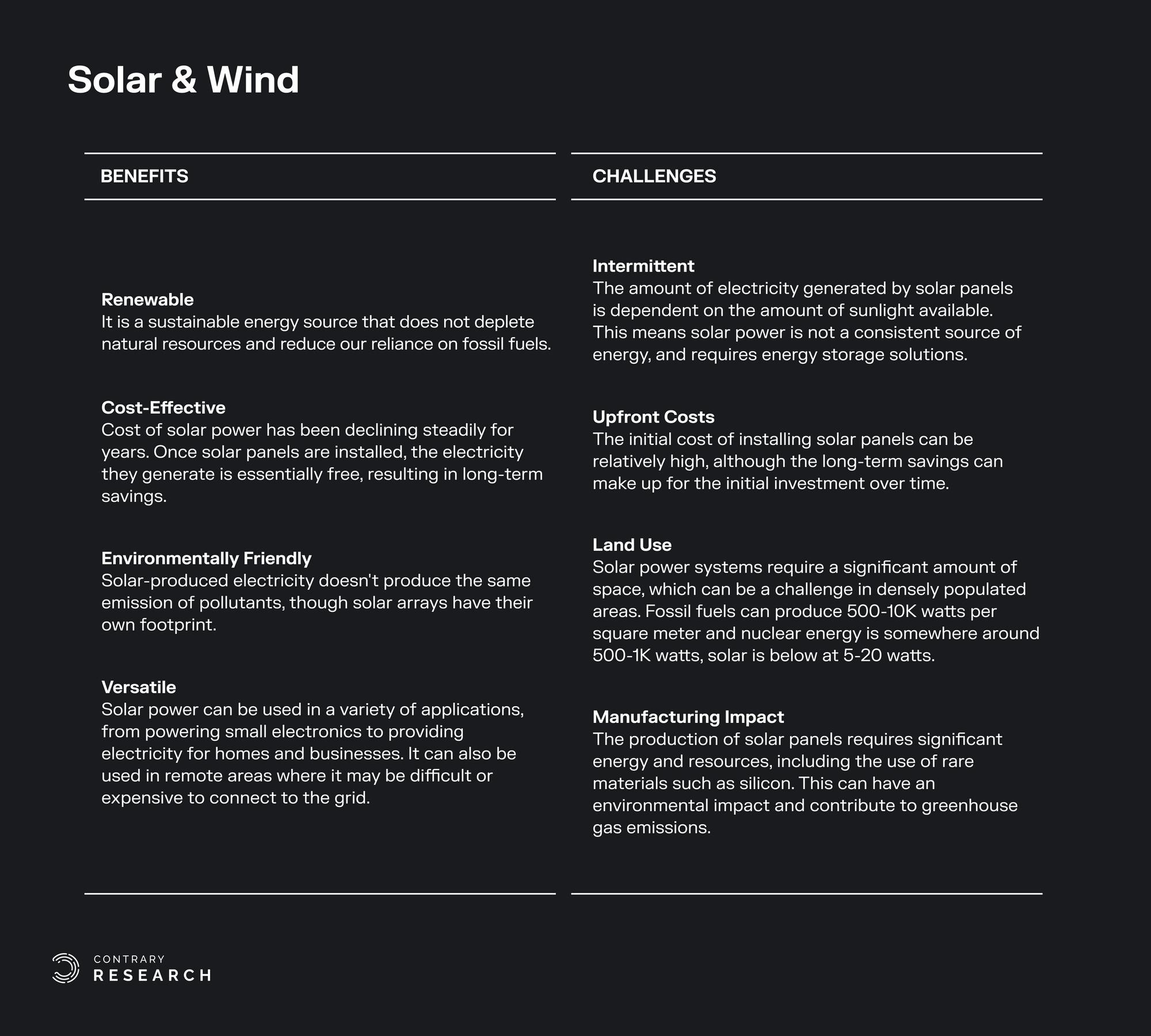
Source: Contrary Research
Another key obstacle for both solar and wind power is often referred to as the duck curve. Energy consumption gradually grows from 6am onwards and peaks from 5pm to 6pm as people come home. Energy from solar and wind surge during the day when sunlight and wind is abundant, often producing more energy to the grid than it needs during the day.
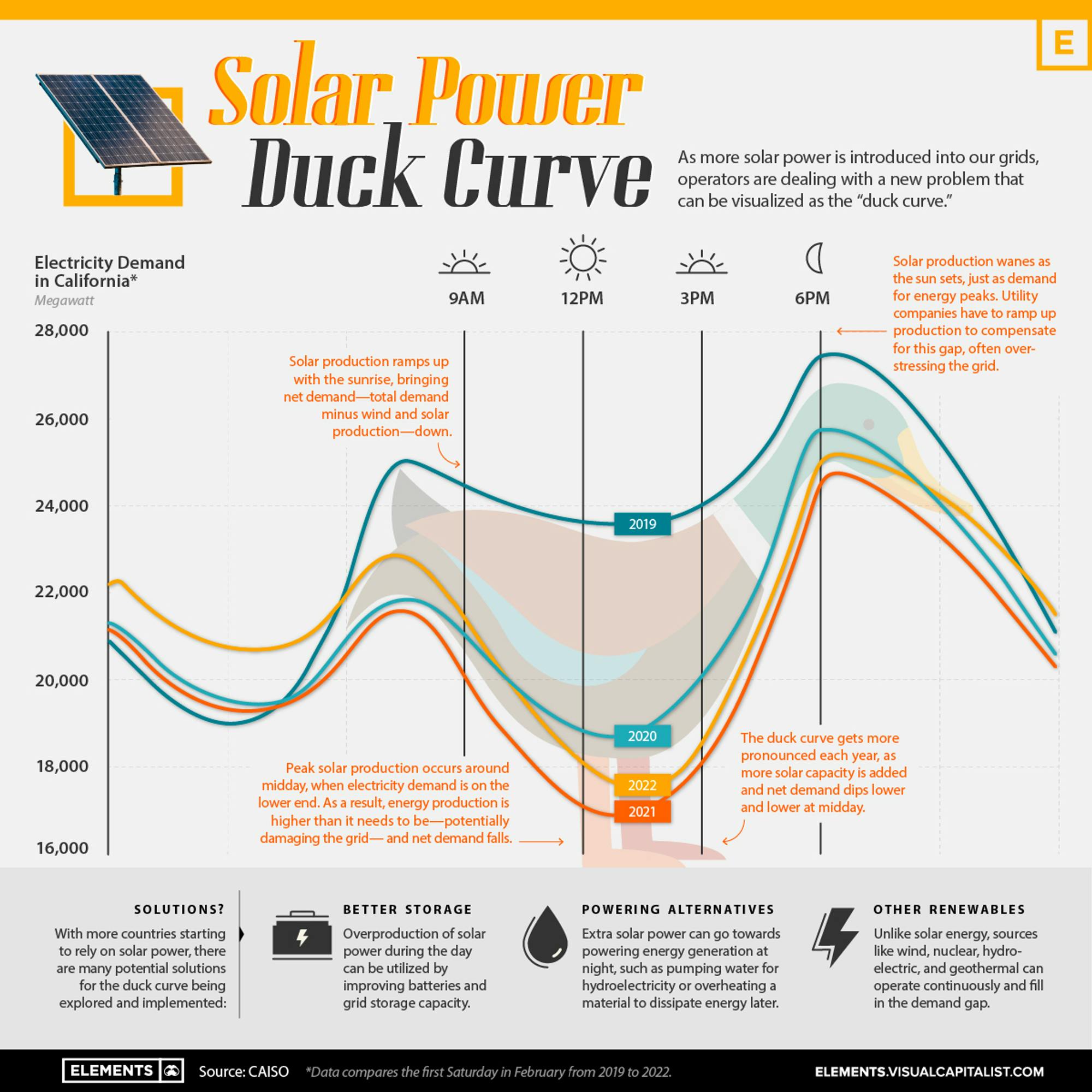
Source: Visual Capitalist
Energy demand begins to surge typically at the same time of day as the sun goes down and wind speeds decline. As a result, utilities are forced to quickly ramp up fossil fuel combustion plants to fulfill net new energy demand as renewable energy availability declines. Until battery and energy storage technology allows us to more effectively store the excess energy during the day or spread it out to lighten the evening load, the amount of solar and wind energy that will be usable in the electric grid will be capped.
While the price of lithium-ion batteries has decreased 97% since 1991, it’s still too expensive to store all of the excess energy from solar and wind. As penetration of renewables increases, the cost of storage represents a significant obstacle to adoption. Even if you assume that over time the cost of lithium-ion batteries will drop by a third, if we got to the point where 50% of energy was coming from renewables, it would cost $49 per megawatt-hour. At 100% that cost jumps to $1.6K per megawatt-hour.
For a deeper exploration into the world of solar power, and battery technology, check out The Silent Rise of Solar Power, The Challenge of Building Better Batteries, and Modernizing the Electric Grid, a series of essays published in Foundations & Frontiers by Anna-Sofia Lesiv.
Hydropower
While advances in solar and wind have been significant over the last few years, hydroelectric power has been a steady and reliable contributor to renewable energy for a long time. While solar and wind emerged in the 19th and 20th centuries, people have been powering things with water for a long time. As of 2022, hydro-generated electricity provided ~16% of the power supply globally, 3x more than wind and 6x more than solar.
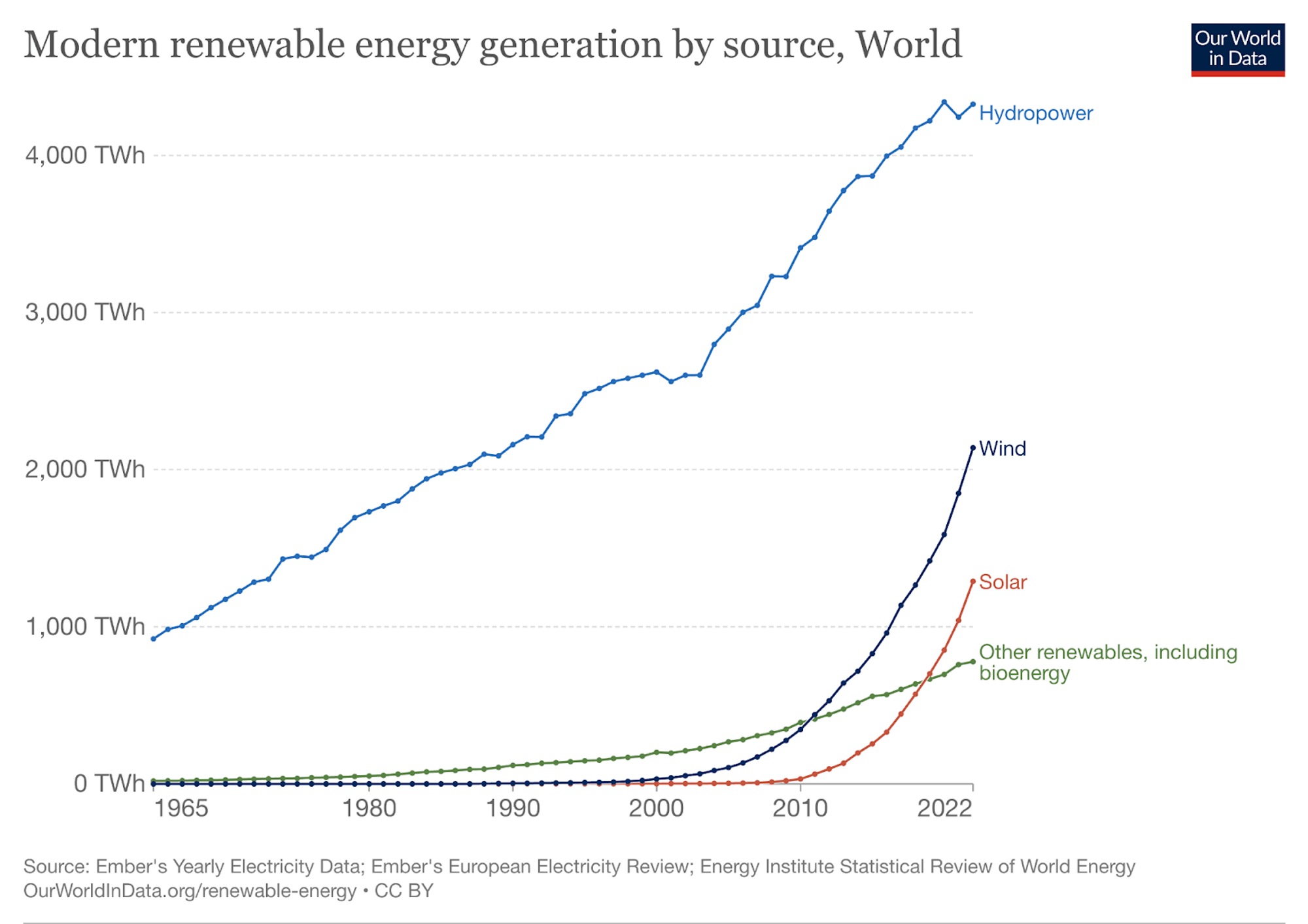
Source: Our World in Data
However, similar to solar and wind, there are also some downsides and limitations with hydropower. There are very few locations on earth that can support a sizable dam to generate hydropower. In addition, dams change the flows of water and dramatically impact areas where people are living. Summarized below are some of the key benefits and challenges that apply to hydropower.
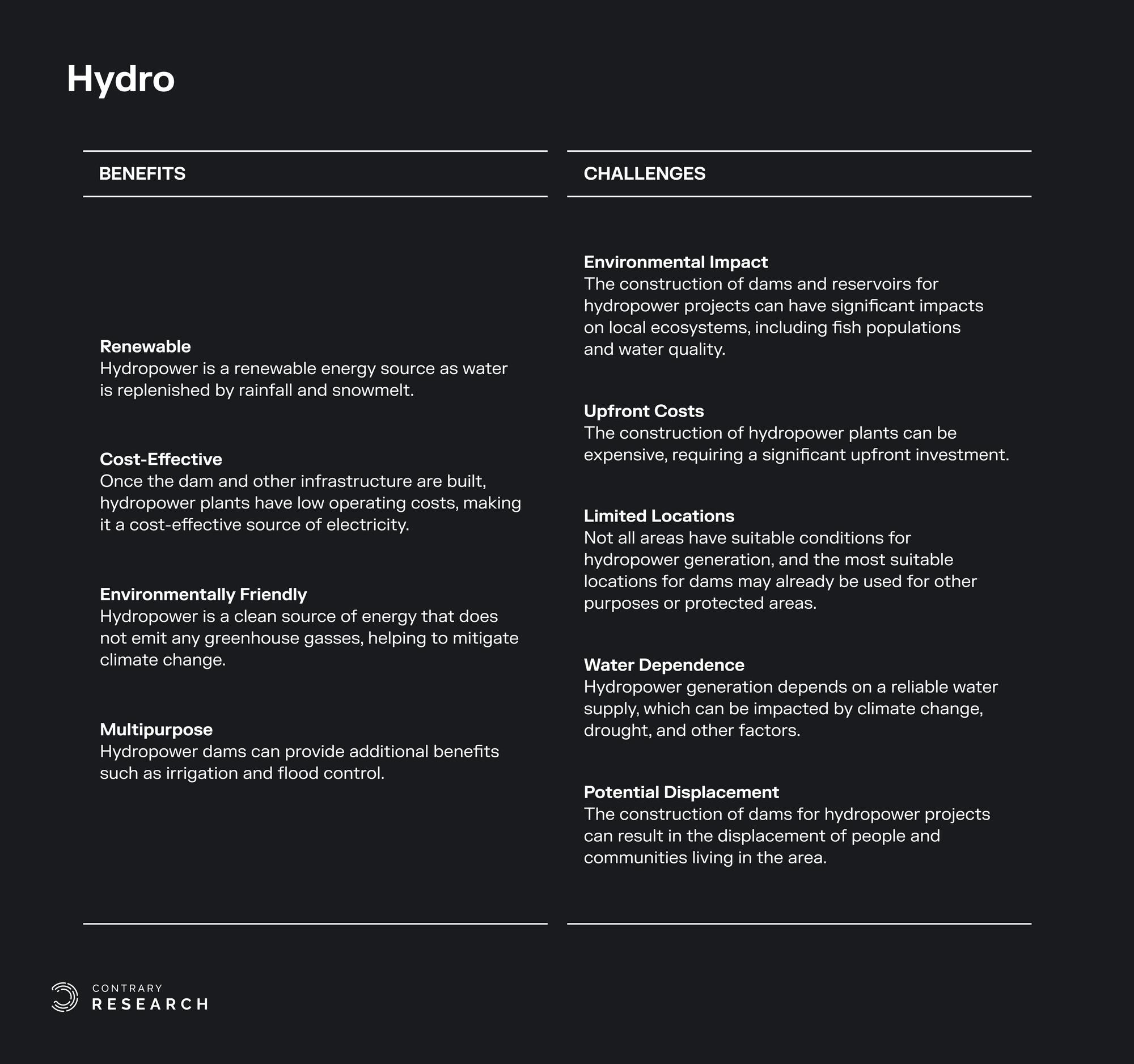
Source: Contrary Research
Nuclear Fission
Nuclear energy has had a controversial history relative to other renewable sources of energy. After disasters like Three Mile Island in 1979 and Chernobyl in 1986, ~50% of people opposed the use of nuclear energy. That opposition put a stop to the expansion of new nuclear facilities, which had nearly doubled from 1978 to 1988. Since then, the number of facilities globally has plateaued.
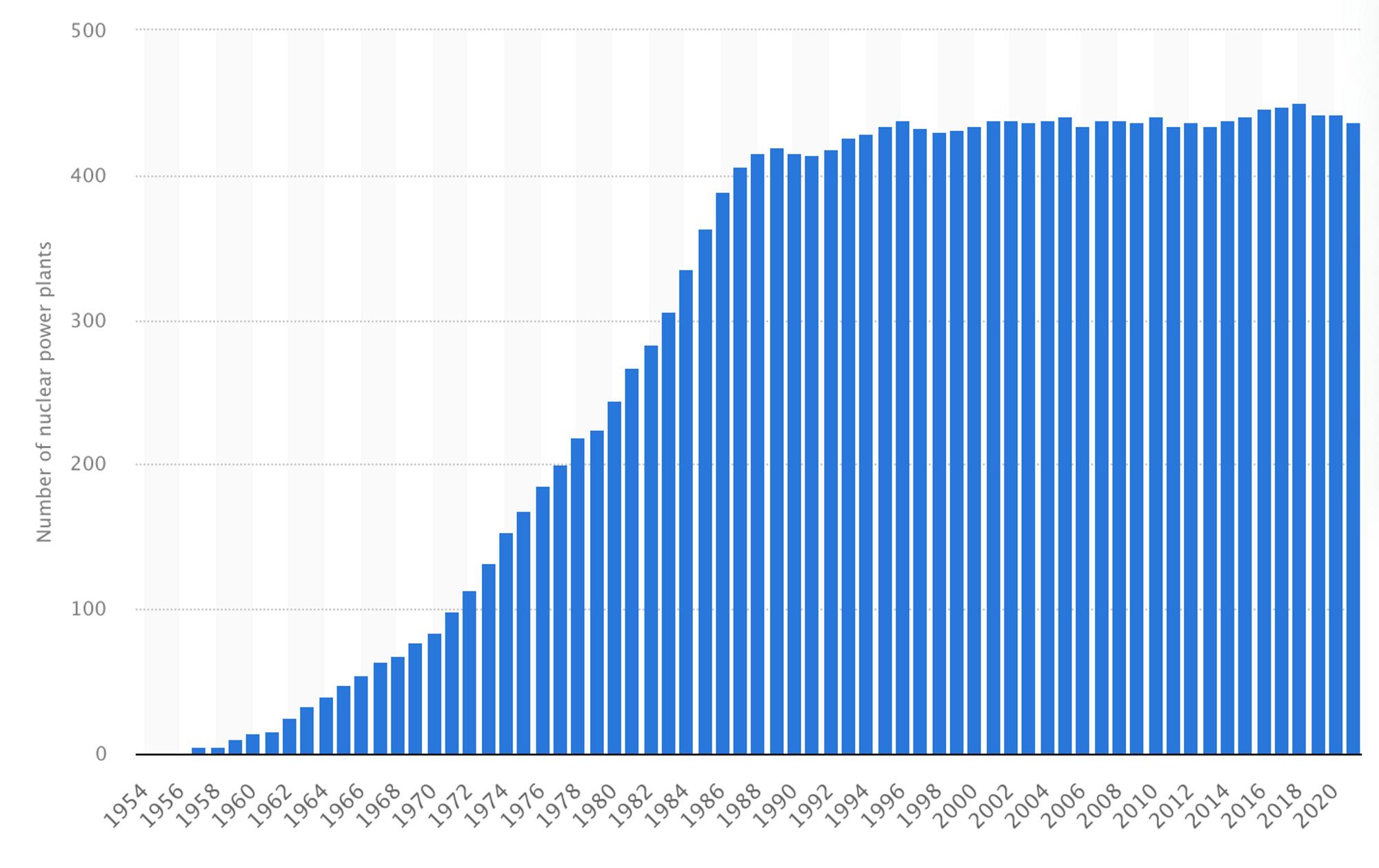
Source: Statista
However, despite additional incidents like Fukushima in 2011, public sentiment around nuclear energy has been steadily improving. Whereas before, in the 1980s, 50% of the US had been opposed to using nuclear energy, as of June 2023, 86% believed nuclear energy will be important in meeting electricity needs in the US in the coming years, and 71% said the US should “definitely” build more nuclear plants.
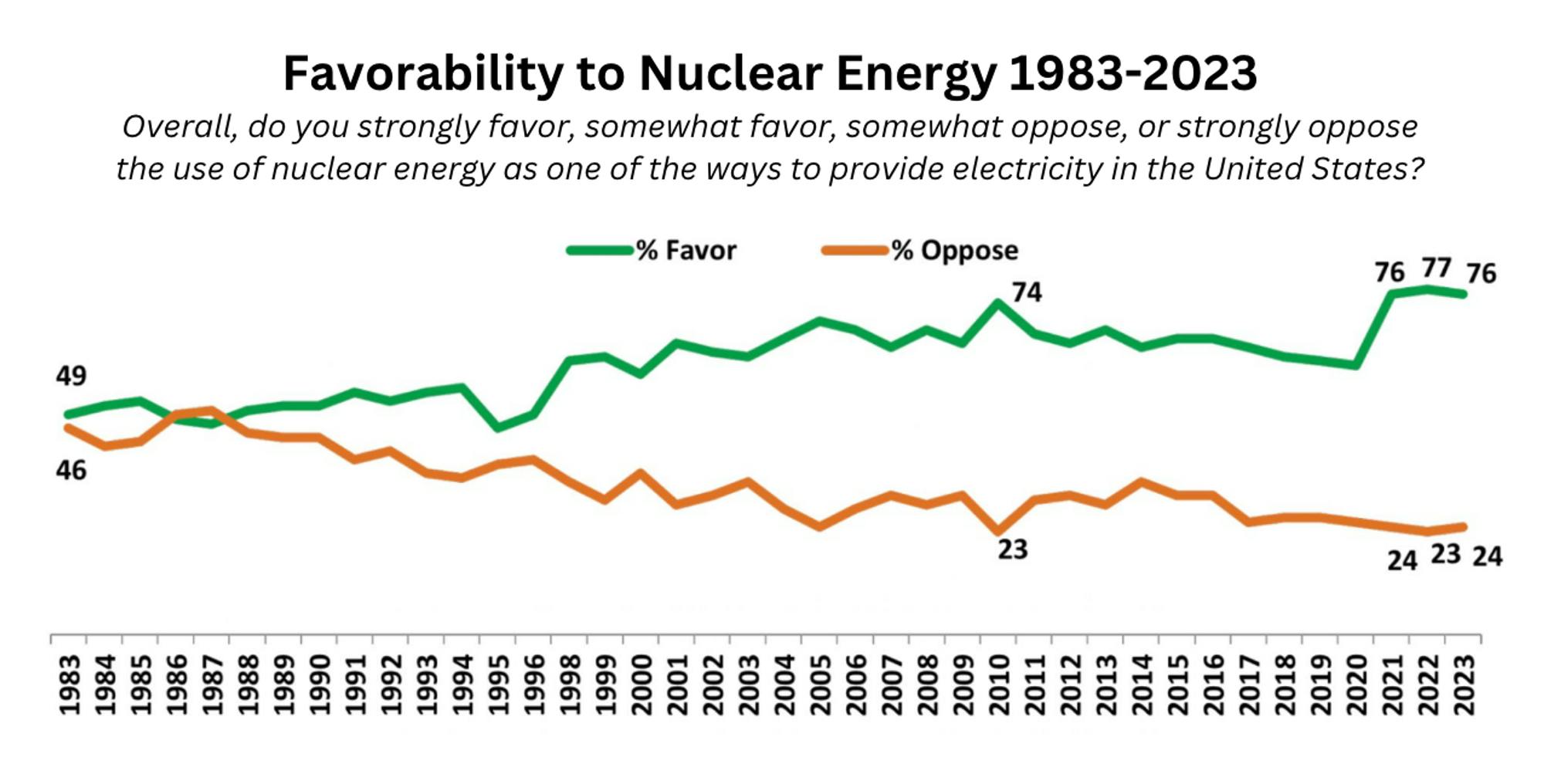
Source: NuclearNewswire
Public opinion has historically posed an obstacle to nuclear energy adoption, general sentiment is continuing to improve. But the technology’s potential has never been in question. Nuclear fission is 8,000x more efficient than fossil fuels. So why aren’t nuclear plants being built left and right?
One element is the cost. In July 2023, Georgia Power announced the opening of Plant Vogtle, the first nuclear reactor built from scratch in over 30 years. However, the project was completed seven years later than expected, and $17 billion over budget. Cost overruns like this can wipe out any future benefits of cost-savings compared to other forms of electricity generation.
Another element is the number of plants that would be required to completely satisfy global energy demand. As of May 2023, there were ~440 nuclear power reactors across 32 countries, with another 60 reactors under construction. One estimate from 2011 projected that to satisfy global energy demand would require 15K nuclear plants.
Some argue that the cost and length of time it takes to build nuclear reactors makes it unlikely that nuclear energy production will be able to keep up with the rapidly decreasing costs of solar and battery storage. However, some countries have seen success with nuclear energy. In France, 70% of the country’s energy supply comes from nuclear energy as of July 2023, although in August 2023 France was forced to cut some of its nuclear energy production as heat waves have warmed the river water that France’s plants use to cool their reactors.
Below are some of the key benefits and challenges that apply to nuclear fission:
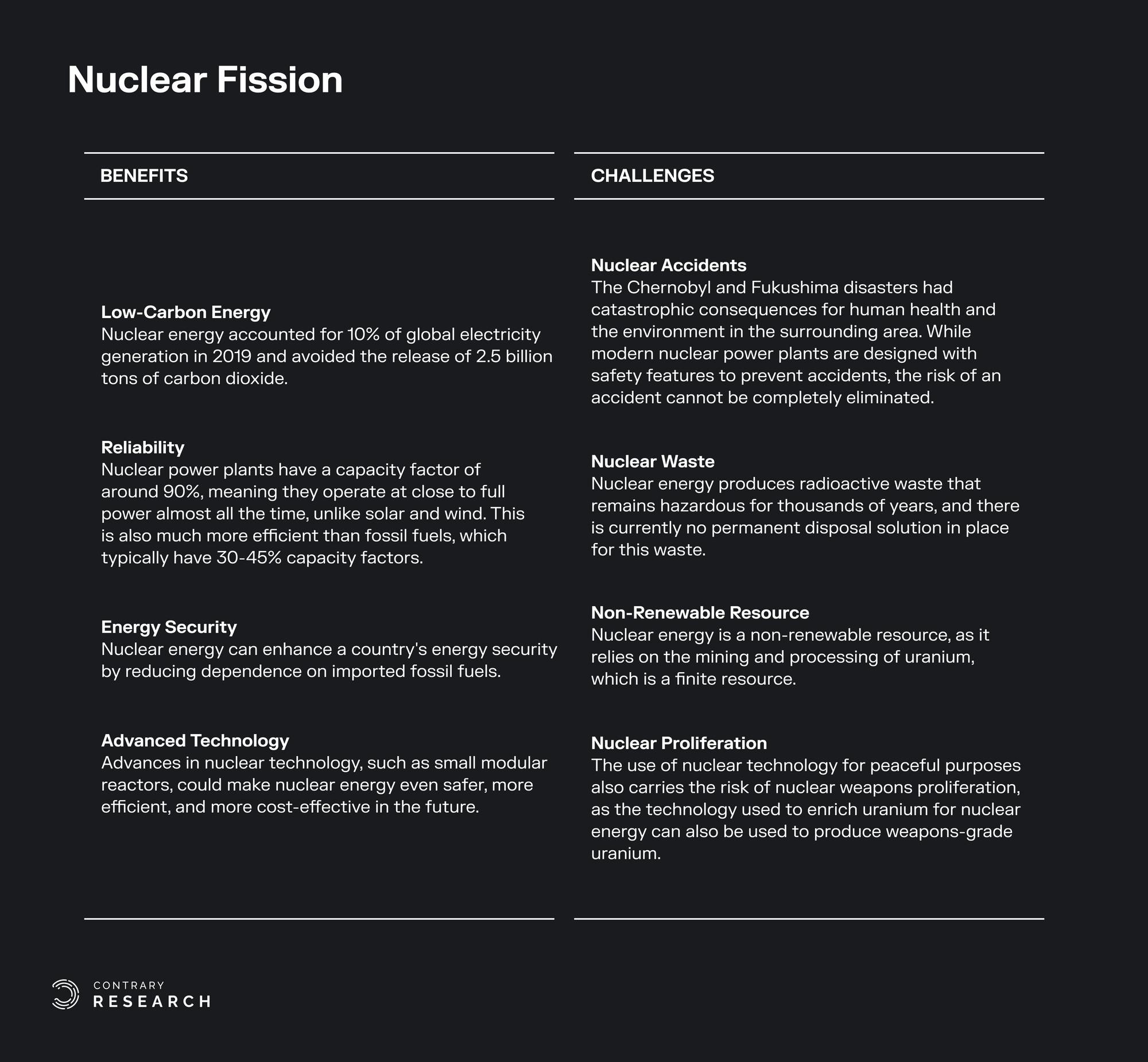
Sources: Contrary Research; IEA, EIA, EPA, Synapse Energy, Proest
The operational complexity of producing nuclear reactors is another significant limitation. Typically, nuclear power plants are decommissioned after 50 years, but at the current rate new plants are being built every 20 years.
Small modular reactors seem to be the most viable solution for nuclear fission moving forward. Companies like BWXT are building microreactors that can fit on a truck bed. The difference in scale is important to note – for instance, each Vogtle plant in Georgia will produce 1.1K megawatts. In comparison, a microreactor will be able to produce between 1-20 megawatts. The Vogtle plants cost over $30 billion to finish, which was twice the initial cost estimate and the state will get about 2.2K megawatts. If a microreactor can reach 20 megawatts and not go over a $300 million budget, that would represent a cost of $15 million per megawatt at best, compared to $27 million per megawatt for the Vogtle plant.
Nuclear Fusion
Historically, energy from nuclear fusion has been seen as the holy grail of energy production. It promises cheap and abundant energy if it were to ever become commercially viable at scale, but the technology for it did not exist until very recently.
Nuclear fusion is the combining of two atoms to produce energy. The theory behind it has been understood for 90 years, but creating a stable nuclear fusion reactor hasn’t been technologically possible. Unlike nuclear fission, fusion is much safer, and the necessary ingredients are more attainable. From a cost perspective, nuclear fusion could be one of the cheapest possible sources of energy generation.
In December 2022, the Lawrence Livermore NIF achieved net energy gain through a fusion reaction for the first time. While small amounts of energy have been created from fusion reactions in the past, the resulting energy creation had always been less than the energy that had to be input. The NIF, however, used 2.05 megajoules (MJ) of energy to create 3.15 MJ, a 50% increase in energy.
While the chemical process may be getting closer to net energy gain, the overall fusion process (e.g. powering the lasers involved) is still too costly in terms of energy to constitute a viable source as yet. Some of the key benefits and challenges that apply to nuclear fusion are as follows:
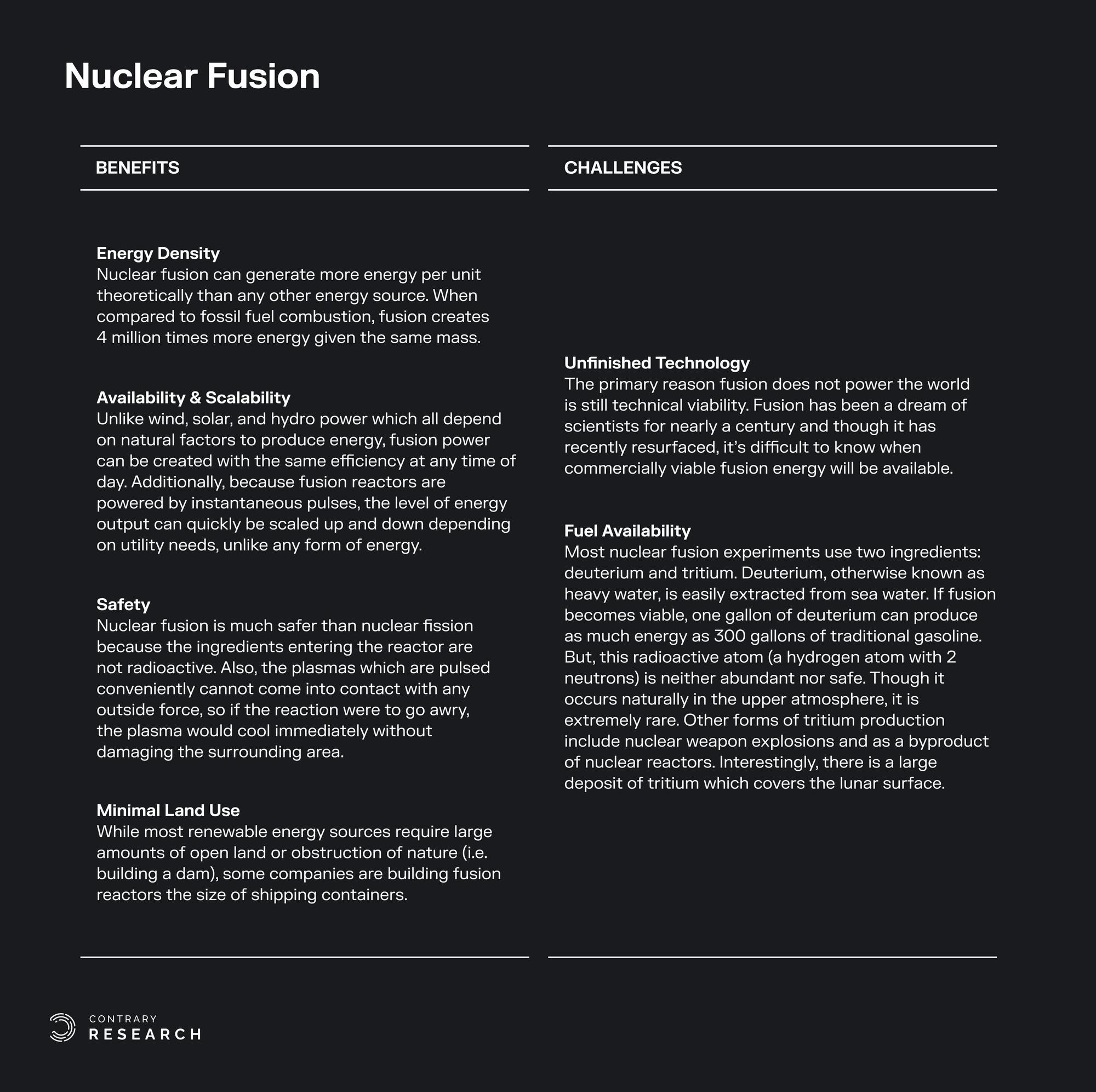
Sources: Contrary Research; ITER, Science Learn, Euro Fusion, ScienceDirect, EPA
For a deeper exploration into the world of nuclear fusion, check out The Promise of Fusion, an essay published in Foundations & Frontiers by Anna-Sofia Lesiv.
Decarbonization Policy Tailwinds
A significant portion of policy historically has addressed specific sources of emissions, like cars with internal combustion engines (ICE). Government programs have increased the adoption of electric vehicles, with electric cars making up nearly 10% of car sales globally in 2022, up more than 60% year-over-year, while growth in overall car sales was roughly flat.
However, as helpful as the transition to electric cars can be in fighting emissions, ultimately those cars will be powered by the electrical grid. And if the grid isn’t being served by renewable energy, the impact is somewhat moot. In fact, regulation like California’s 2035 ICE car ban may put an undue strain on the grid if it isn’t able to manage the additional demand from car charging.
For a deeper exploration into the world of the electrical grid, check out Modernizing The Electric Grid, an essay published in Foundations & Frontiers by Anna-Sofia Lesiv.
Rather than addressing symptoms, a number of government policies are attempting to address the core disease and help push different organizations towards a decarbonized future. Some of those policies include:
Renewable Portfolio Standard (RPS): Requires utilities to generate a certain percentage of their electricity from renewable energy sources. Examples include California (100% by 2045) and New York (70% by 2030).
Solar Parking Lots: Requires all parking lots larger than 2.5K square meters (over 80 parking spots) to be covered in solar roofing. France created this policy in November 2022, possibly generating as much energy as 11 nuclear reactors.
Feed-in Tariff (FIT): Guarantees a fixed rate (usually above market price) for renewable energy producers. Germany implemented this policy in 2000, and as a result, it has become the global leader in solar power.
Net Metering: Allows consumers with solar panels to sell excess electricity back to the grid. In the US, 41 of the 50 states allow for net metering. In 2015, Hawaii ended net metering for all subsequent solar installations due to excess energy supply.
Renewable Heat Incentive (RHI): Provides financial incentives to homeowners and businesses that switch to renewable heating systems. The UK implemented this policy in 2014.
Carbon Pricing: Places a price on carbon emissions to incentivize companies to reduce their greenhouse gas emissions. The European Union Emissions Trading System (EU ETS) is an example of this policy.
Tax Credits: Provides tax incentives for investments in renewable energy. The United States implemented the Investment Tax Credit (ITC) in 2006 to support the development of solar and wind energy, causing an average annual growth rate of 24% for solar in the US.
Green Bonds: Allows governments to finance renewable energy projects through the issuance of bonds. France has issued green bonds to finance countless renewable energy projects.
Energy Efficiency Standards: Requires appliances and buildings to meet energy efficiency standards. The European Union has implemented this policy, mandating all countries increase energy efficiency yearly by 0.8%.
Zero-Emission Vehicle Mandate: Requires a certain percentage of vehicles sold in a state or country to be zero-emission vehicles. California and several European countries have implemented this policy.
Renewable Energy Targets: Sets a specific target for the amount of renewable energy to be generated in a country. India has set a target to generate 175 GW of renewable energy by 2022.
Energy Storage Incentives: Provides financial incentives for the development of energy storage technologies. Germany and the United States have implemented this policy to support the integration of renewable energy into the grid.
Smart Grids: Enables the integration of renewable energy into the grid by improving the management and distribution of electricity. China has invested heavily in the development of smart grid technologies.
These policies are framing the landscape that companies will have to operate in as they decide what their carbon footprint will be. With these types of policies as a backdrop, we can start to unpack what the future may hold for decarbonization efforts.
Powering The Future
Voluntary Carbon Markets
The future of decarbonization will include both compliance and voluntary carbon markets. We touched earlier on the compliance carbon markets where companies will be forced to comply with various regulations around emissions management. The voluntary carbon market represents companies that aren’t required by law to achieve statuses such as carbon neutral, but they attempt to anyway. Some estimates put the size of the voluntary carbon market at $2 billion in 2021, quadrupling from 2020. Those same estimates indicate that the voluntary market could reach between $10 biillion and $40 billion by 2030, while still others expect that number could reach as high as $50 billion.
The amount of voluntary offsets increased 2x between 2020 and 2017, and the number of companies with net-zero targets doubled from 2019 to 2020. But for growth to continue in voluntary markets, certain changes in the future will have to occur, both to address skepticism of the category and to improve the quality of the practice.
Increasing Supply of Carbon Offsets: Certain types of credits such as nature-based credits (reforestation, blue carbon, soil and agriculture) will soon see demand exceed supply. In addition, companies are increasingly interested in purchasing offsets created by removal credits, rather than avoidance credits (which make up 80% of supply today.)
Monitoring, Reporting, & Verification (MRV): To prove additionality, credits can use MRV to track the affected area as well as areas not affected by offsets. More than 90% of buyers rank MRV as a major criteria for purchasing credits to ensure that the credits they buy have an impact that can be easily proven and defendable against claims of greenwashing.
Additional Regulation Around Offsets: Today carbon markets are fairly opaque. Additional regulation could help define which types of emissions are avoidable, what percent of emissions can be offset vs. reduction efforts, and more.
While there is significant room for carbon markets to improve, the reality is that there is still a significant imbalance between the volume of demand for offsets and the supply of high-quality offsets. Goals like those set out by the Paris Agreement won’t be reached unless additional efforts are made towards carbon emissions reduction and carbon removal. Even with carbon removal, there is still a critical need for carbon reduction given that one ton of carbon removed from the atmosphere is not equivalent in terms of its impact on climate to one ton of carbon emissions avoided.
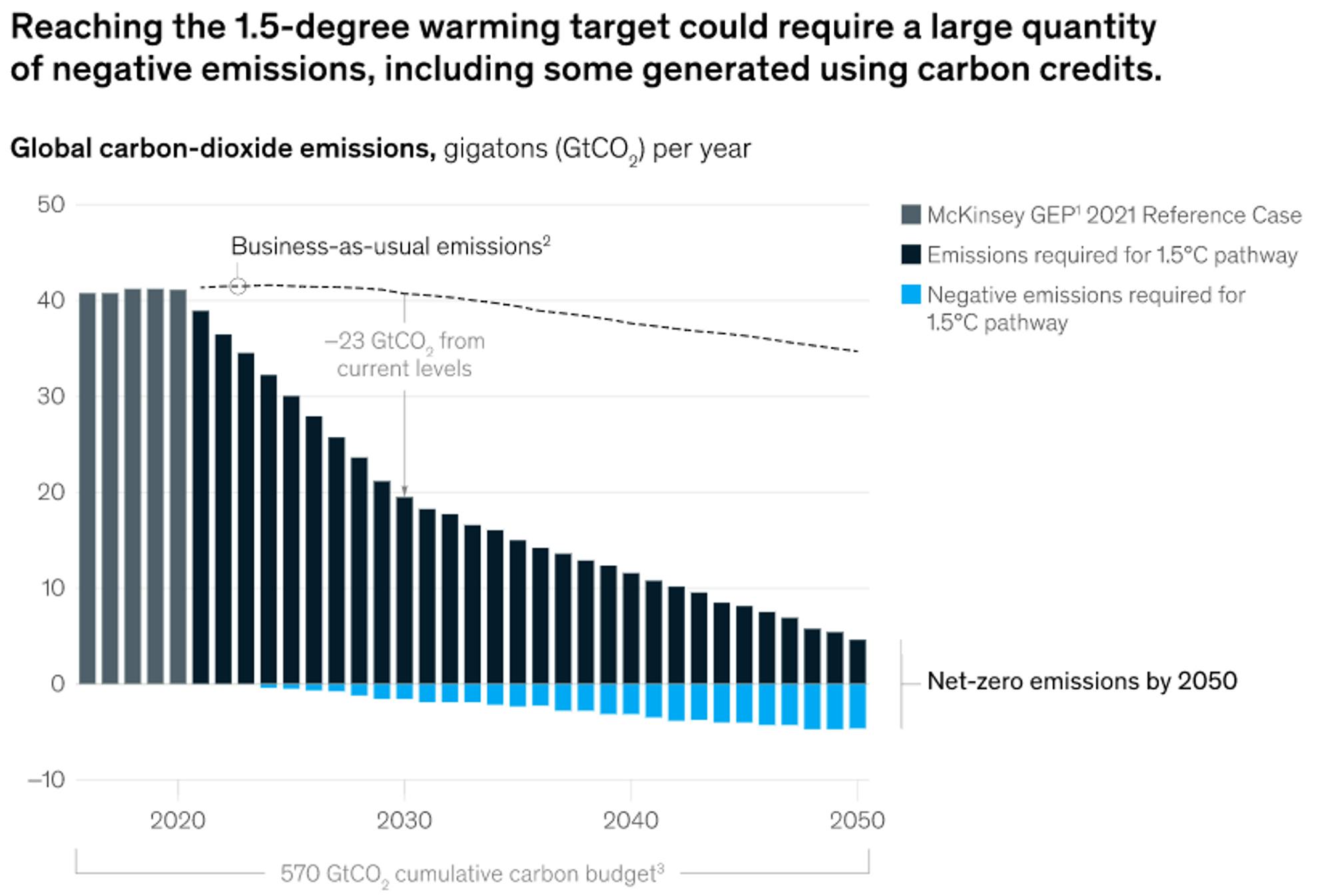
Source: McKinsey
A critical part of carbon emission reduction will come from the increased adoption of renewables across a broader set of use cases.
Increased Adoption of Renewables
Solar and wind, which were estimated to see modest growth from 2006 to 2030, have seen rapid growth instead. One estimate indicates that by 2035, 60% of the world's energy could come from renewable sources. From 2020 to 2026, renewable energy production could increase by 80%, primarily driven by solar and wind power. These alone could account for over a third of global energy production by 2030. 95% of net-new power capacity over that same time period is expected to come from renewable sources.
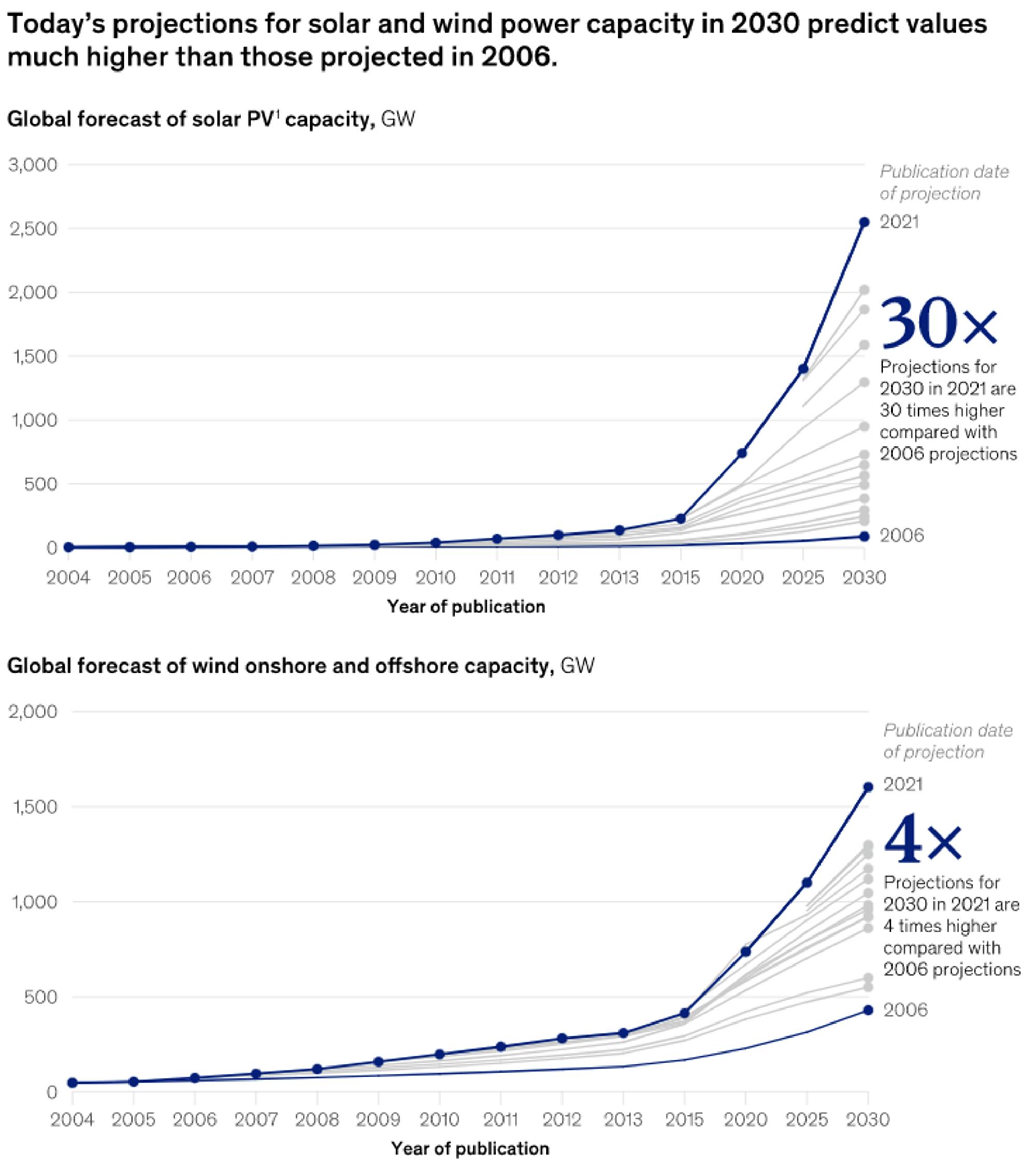
Source: McKinsey
A number of proponents of renewables have continued to make investments, including some unexpected participants:
Oil and gas companies are shifting business models to capitalize on green energy and EVs.
Private equity and institutional investors are leveraging government incentives and high demand to make profitable investments in renewables, especially at scale.
The shipping industry is pushing for production of hydrogen and ammonia as clean fuel sources.
Steel manufacturers are targeting green hydrogen to decarbonize steel production.
As of 2021, China led the world in volume of renewable energy capacity added, followed closely by India, which has doubled its new renewable installations since 2015. These countries, along with Europe and the US, account for 80% of renewable expansions worldwide. While positive tailwinds exist for renewables, there are still a number of things that still need to happen.
Increased global access to renewables: renewable energy needs to be more directly integrated into the grid, not just for the wealthiest communities. Countries with high emissions also need to take responsibility for their outsourced emissions, like when poorer countries produce higher emissions to manufacture goods for wealthier countries.
Domestic policies around the world: while countries in Europe, the US, and China are making some proactive efforts towards renewables, it isn’t enough. Reducing market risk and incentivizing investments (often in the form of removing red tape) through policy are essential. For 1.5C targets to be reached, the share of renewables in global electricity generation must increase from 29% to 60% by 2030. That will require additional countries to take similar measures.
Advances in subsidies: in 2020 alone, $5.9 trillion was spent on subsidizing the fossil fuel industry. These include explicit subsidies and tax breaks but also include health and environmental effects that are not priced into the cost of fossil fuel, which is about $11 billion every day. These subsidies are one of the largest reasons fossil fuels are still a good option for many processes over renewables. There is also a question of equality within these subsidies as well. For developing countries, half of these subsidies only benefit the top 20% of the population.
Significant additional investments: to reach 2030 intermediary goals, investments in renewable need to reach $4 trillion per year until 2030, or 2050 goals will most likely become out of reach. Interestingly enough, that is only two-thirds of the annual subsidies to fossil fuel companies listed above. Additionally, these subsidies have positive side effects such as depolluting the air which could save the world an estimated $4.2 trillion per year.
While the shift towards renewables feels inevitable, there are also some critical obstacles standing in the way:
Poor grid infrastructure: the electrical grid is not effectively designed to handle the integration of renewable energy sources, which are intermittent in nature. A utility’s primary job is to balance supply and demand, which is made harder by the volatility of solar and wind. Additionally, the grid wasn’t made to optimize for energy outflows and inflows from different units. Some proportion of these problems can be solved by smart grids which allow for real-time supply and demand management and two-way communication among other things like increased data transparency.
Land use: while roofs are prime spots for solar panels, finding land that is cheap enough to turn a profit that also complies with regulations can be difficult. For example, Germany is trying to increase onshore wind farms. Of the 51% of German land that was flagged as potentially suitable, regulatory, environmental, and technical constraints reduce this number to a mere 9%. To meet Germany's goals, 4%-6% of its land must be covered by wind farms, so investors have a very small set of options.
Workforce shortages: Between 2022 and 2030, an additional 1.1 million blue-collar workers are needed to construct solar and wind plants, not to mention the additional 1.7 million needed to operate and maintain these systems. 40% of employees say they are likely to leave their company within 3-6 months, which makes filling these roles challenging.
Logistic and supply chain issues: nickel, copper, rare earth metals for magnets in turbines, and more are projected to be in short supply given the level of growth expected for renewable energy production. To avoid supply issues, resource efficiency can be increased to reduce the amount of materials needed per system along with general R&D to find new materials and technologies to create panels and turbines. Lastly, recycling of rare materials from retired systems (i.e. copper from old wind turbines) and reusing of entire systems (i.e. decommissioned solar farms selling solar panels to new developments) will reduce needed materials.
Key policies driving compliance carbon markets, as well as changing sentiment around voluntary markets will be a critical starting point. Renewables will likely be a big part of the equation. But the myriad of solutions that exist will address more than just these categories. The solutions needed over the next 10-15 years will spread across energy creation and storage, scientific progress, sophisticated financing, climate adaptation, and more.
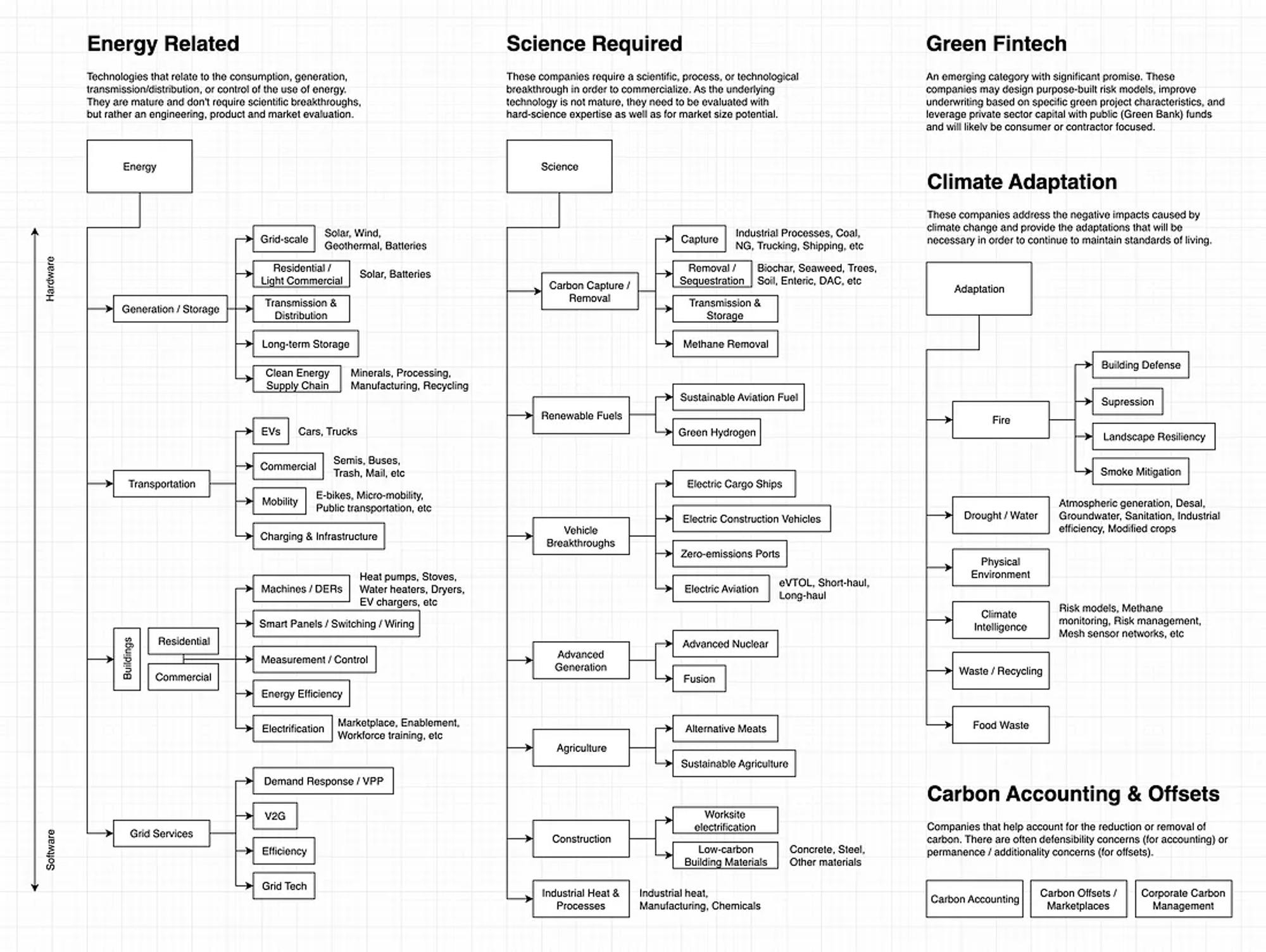
Source: David Rusenko
These high-level categories can be broken down further into sub-categories like energy generation, battery and energy storage, carbon management, precision agriculture, and more. In our Living Landscape: Climate Tech, we break down these categories and the relevant businesses tackling each of them.
However, after mapping out the types of possible solutions, it then becomes a question of timing. Most proponents of addressing climate change agree that, while there are some short-term changes we can make, the real global impact comes from making long-term commitments.
Short-Term vs. Long-Term Energy Needs
Elon Musk, one of the more public and outspoken figures in the fight to end fossil fuel consumption, has a contrarian perspective when it comes to renewable fuel sources. In an interview at the Oslo Oil and Gas Summit in 2014, Musk said the following:
"If there was a button I could press to stop all hydrocarbon usage today, " Musk says, "I would not press it. It would cause civilization to come to a halt. It would be irresponsible to press that button… What does need to happen, if we can, is to accelerate the transition towards renewables."
More recently, in a 2022 interview in Norway, Musk further commented on the timeline for renewable energy transition efforts:
"Realistically I think we need to use oil and gas in the short term, because otherwise civilization will crumble… One of the biggest challenges the world has ever faced is the transition to sustainable energy and to a sustainable economy. That will take some decades to complete."
Moving the world off fossil fuel combustion and onto renewables will take a number of years, but the important thing is making steps every year to reach goals. According to the IEA, there are three main scenarios and respective models for energy usage until 2050.
STEPS (Stated Policies Scenario)
APS (Announced Pledges Scenario)
NZE (Net Zero Emissions)
Each one is progressively more optimistic. STEPS is a bottom-up model of what companies, governments, and institutions are actually doing right now. APS is an estimate based on what governments and companies have pledged and officially announced they are committed to. NZE is what would be required to reach carbon neutrality by 2050.
STEPS: The proportion of global energy related carbon dioxide emissions falls below 75% by 2030 and just above 60% by 2050. The highest point for these emissions occurs in 2025, reaching 37 billion tonnes (Gt) per year, but declines to 32 Gt by 2050. As a result, global average temperatures are expected to rise by around 2.5 °C by 2100. Although this is a more favorable outcome than previously projected, with policy and technological advancements since 2015 reducing the long-term temperature rise by about 1 °C, the reduction of only 13% in annual carbon dioxide emissions by 2050 in the STEPS scenario is insufficient to prevent severe consequences of climate change.
APS: Predicts a short-term peak in annual emissions, followed by a faster decline to 12 Gt by 2050. This reduction is more significant than previously estimated in the WEO-2021 APS, mainly due to recent commitments from countries like India and Indonesia. Additionally, the APS includes commitments from specific industries and companies for the first time, and if all these commitments are met on time, the temperature increase by 2100 would be around 1.7°C. However, it is essential to note that fulfilling these commitments will be challenging, and more action is necessary to align with the NZE Scenario. This scenario aims to reduce yearly emissions to 23 Gt by 2030 and achieve net-zero by 2050, resulting in a 1.5°C temperature increase.
Net Zero Emissions by 2050 Scenario (NZE): A normative IEA scenario that shows a pathway for the global energy sector to achieve net zero carbon dioxide emissions by 2050, with advanced economies reaching net zero emissions in advance of others. This scenario also meets key energy-related United Nations Sustainable Development Goals (SDGs), in particular by achieving universal energy access by 2030 and major improvements in air quality. It is consistent with limiting the global temperature rise to 1.5 °C with no or limited temperature overshoot (with a 50% probability), in line with reductions assessed in the IPCC in its Sixth Assessment Report.
In the path towards meeting decarbonization goals, advancements like solar storage, carbon capture, and nuclear fusion will likely play a big role in the transition to renewable energy. The short term, however, presents a different situation as underinvestment in drilling infrastructure has led to a tightening of oil supply. That was especially relevant as Russia invaded Ukraine. Russia exported somewhere in the ballpark of 8 million barrels of oil per day, which was roughly 8% of global supply. And that caused oil prices to get up to $120 at one point. And that was despite China, the largest net importer of oil, being under strict lockdowns. Now that China has reopened, the back half of 2023 looks trickier as global demand is set to surge.
In a world where renewable energy sources still have a long way to go before there is adequate supply, taking a moralistic stance on energy sources is a luxury only citizens of developed or affluent nations can really afford. It’s easy for such populations to look down on a nation like India for importing 40% of its oil from Russia, but from the perspective of developing countries, it’s not feasible yet to fully implement solar, wind, and nuclear to have a sustainable energy economy.
Evidence points to the fact that burning fossil fuels have increased carbon levels, which directly affects global temperatures. It’s not necessarily the absolute amounts of carbon right now that is concerning, but the rate of carbon emission if the current trajectory remains unchanged. Therefore, governments and corporations around the globe are investing in renewable sources. None of these sources individually are perfect panaceas, but as a group they will continue to grow, improve, and make up a larger chunk of the global economy in a decarbonized, sustainable future.